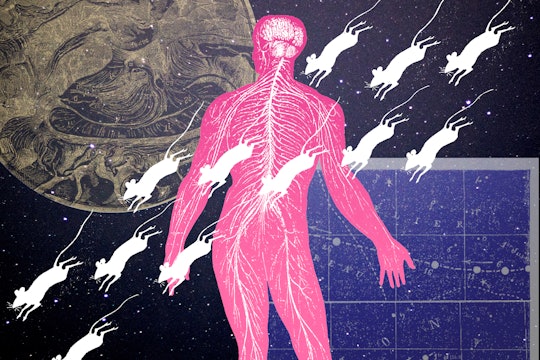
Want to learn how brains go awry? First, we must learn how they work
We set out to make neurons deep in the brain glow bright so we could see their role in vision
I still vividly remember the day in 2013 when Dr. Euiseok Kim, a postdoctoral researcher at the Salk Institute, stepped into the lowly graduate student office to discuss his project. It was like when Nick Fury goes into some corrupt neighborhood to pull out yet another unknown-but-actually-badass superhero to recruit for The Avengers. “We’ve got a mission, and we need you, Ashley.”
OK, so maybe it wasn’t quite so dramatic.
In any case, here was the plan: Euiseok had identified genetic markers for three different neurons, or brain cells, located in the brain's visual cortex, a region that’s incredibly important for sight. The neurons all had slightly different shapes and connections to other brain areas. Euiseok wanted to find out whether they had different roles in the visual system. And he wanted me to help him do it.
Our team — like The Avengers, but far nerdier — set our sights on determining the function of these cells. First, we needed to find a way to mark these neurons and make them look distinct so we could watch them work. If we want to know how all of these neurons ultimately fit back together and sometimes go awry, we needed to be able to study them in isolation.
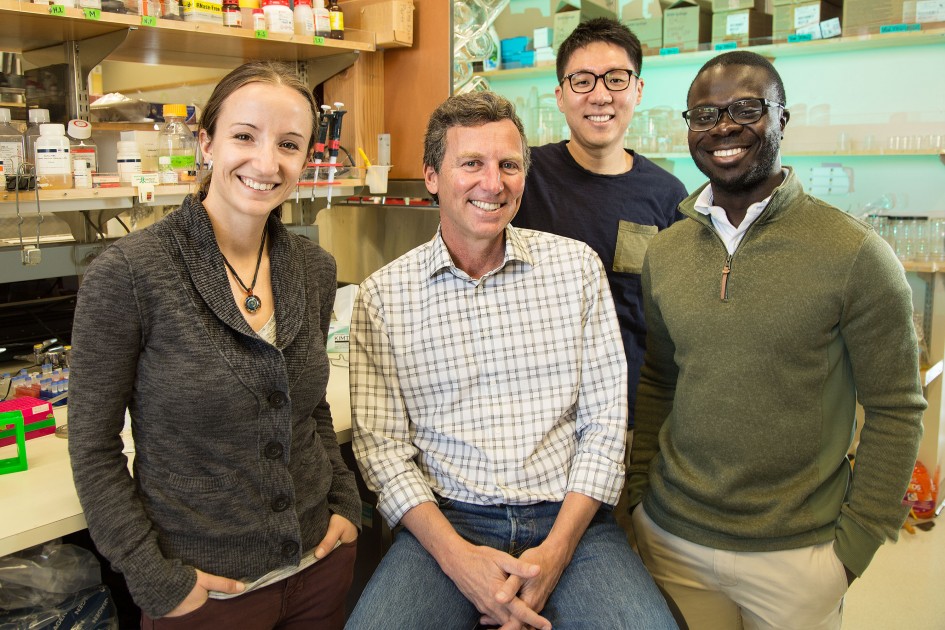
From left to right: Ashley Juavinett, Ed Callaway, Euiseok Kim, Espoir Kyubwa
Photo via Salk Institute for Biological Studies
But we can’t exactly actually tease apart a human brain and pull out individual neurons. They're packed in like sardines. So, many neuroscientists study the mouse brain instead. And instead of pulling out neurons, we peered in, using the combined power of genetics and light.
We engineered some mice to express a protein called “Cre Recombinase” in a specific set of cells. Cre Recombinase acts like a key that can unlock proteins, including ones that glow. You can introduce these proteins into a mouse as a virus, which acts like a protective package. When the virus infects the neuron and Cre Recombinase is present, it unlocks all of the useful proteins inside it.
With this strategy, we made each of our neurons of interest glow. Even better, they glowed differently based on how much calcium that was flowing into the cell, a decent estimate of cellular activity. More activity, more calcium, more brightness. We then used lasers to specifically illuminate tiny parts of the brain so that we could see this change in activity, a technique known as two-photon calcium imaging.
While many labs have introduced glowing proteins into mouse brains in order to study activity, few labs have been able to do this so deep in the cortex. The first few times I tried, I couldn’t see anything. We had to be innovative, adapting our techniques in order to be able to see about half a centimeter down. This meant using more laser power, making sure the brain was really healthy, and installing a glass window in place of the skull that would keep everything intact.
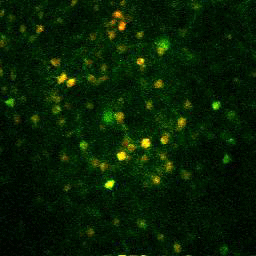
Calcium imaging in action.
Photo by Ashley Juavinett, Euiseok Kim, Edward Callaway
I waited patiently, tweaking multiple parameters to make our method work. I spent hours in front of the computer, searching around the brain like an astronaut searching for a planet in a dark, endless sky. And then suddenly, there it was: the first gorgeous, glowing neuron. I gaped at it for several minutes and then ran to get our advisor, Dr. Edward Callaway. I wouldn't normally interrupt him — he’s a top investigator at a fancy palace of science — but this was worth it.
After that, we found many more neurons. We showed the mouse different visual stimuli on a monitor while imaging its brain. With enough cells of each type, we were ultimately able to say that indeed, they were functionally different. Neurons that send connections deep into the brain care about things that are moving, likely because these are the cells involved in helping us initiate movement and navigate around in the world. On the other hand, neurons that talk to other cortical areas seem to care more about detail, likely because these are the cells involved in object recognition. We had successfully proven that these cell types could be distinguished based on a few genetic markers, and that they had different functions in the brain.
Now we can use this information to design additional studies that dive deeper into the cells' role in vision — and beyond. Sensory disorders such as schizophrenia and autism likely rely on the proper functioning of these cell types. With the ability to identify and classify at least a few different types, we can start figuring out whether they’re responsible for problems with sensory processing. The next generation of lab avengers is diving even deeper, trying to understand how these cell types communicate with nearby cells.
These are fundamental questions that we need to answer if we want to know how the brain works, and what happens when it doesn't.
Featured Article
- Kim. E.J., Juavinett, A.L., Kyubwa, E.M., Jacobs, M.W., Callaway, E.M. (2015). Three Types of Cortical Layer 5 Neurons That Differ in Brain-wide Connectivity and Function. Neuron 88(6): 1253-67. https://doi.org/10.1016/j.neuron.2015.11.002