Chapter 3
(Deli) Counter Culture
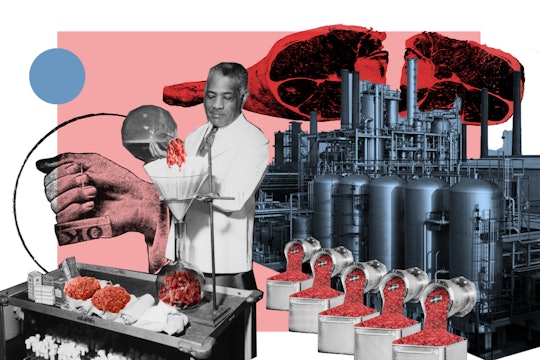
Allan Lasser
What is cultured meat?
Cultured meat may be a safe and sustainable alternative to slaughtered meat. It's made by cultivating cells in laboratories. These cells are then used to make sausages, hamburgers or 'nuggets'. The possibility of creating more structured cultured meat products, such as steak, chicken breast or salmon fillet is still an open field of research.
What do NASA and PETA have in common? They have both said that people need to find a way to eat meat without animal farming and slaughtering. Industrial livestock has negative health and environmental impacts. But luckily for carnivores, researchers have been working on cellular agriculture, which aims to combine biotechnology with food and tissue engineering to produce meat from cell cultures in a lab.
Since the first cell burger trial in 2013, the cultured meat community has been slowly growing. And the idea of growing meat is certainly not new. In Kurd Lasswitz's 1897 novel Two Planets, Martians introduced “synthetic meat” on Earth. In late 1931, Winston Churchill wrote in an essay, “We shall escape the absurdity of growing a whole chicken in order to eat the breast or wing, by growing these parts separately under a suitable medium.” But it wasn't until around 2000 that there were real attempts to grow muscle cells in a lab—they involved edible goldfish muscle as food for space travelers.
Today, many companies, research institutes, and non-profit organizations are developing cultured meats and fish products—but what exactly is cultured meat?
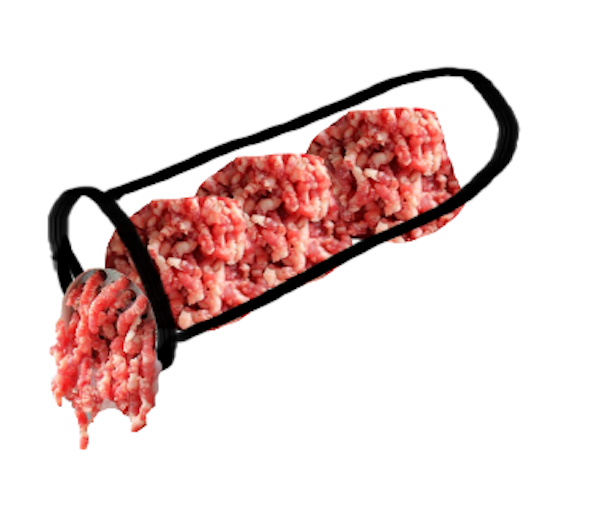
Cultured meat will hopefully look and taste like conventional meat from animal tissue. The difference is that the cells making up cultured meat are not grown inside of an animal. Instead, the cells are allowed to grow in culture flasks or larger systems for growing individual cells in a lab. If those cells are organized, then the product becomes a structured meat—like a steak or a chicken breast. To give them structure, edible scaffolds are added as the foundation for cells to grow on, mimicking the natural architecture and appearance of cuts of meat. Alternatively, it's also possible to grow unstructured cells, which mimic ground meat products like sausages, hamburgers, and chicken nuggets.
The future industrial production of these meats will require "farms" for cellular agriculture. Instead of housing cattle, the farms will be a place to grow cells, assemble them into meat products, and store and vacuum-seal them for quality. This type of facility is still on the wish list for producers because cultivation and harvesting processes are still being optimized. Medium-sized meat cultivators may be an alternative to blend in with the livestock industry that can be used along with traditional farming practices. Cultured meats might also be produced at home with smaller devices, as some initiatives seem to be encouraging.
Lab-grown meat products will be deeply studied to ensure their safety, and to be continuously tweaked to taste and look like conventional meat. At the same time, there is plenty of room to design innovative cultured meat products that are different from conventional meat, like one cultured meat company, Modern Meadows, invention: meat potato chips. There is an increasing hunger out there for meat in just about any form, so why not in chip form?
How do we make cultured meat?
Like conventional meat, cultured meat is composed of animal cells. Instead of slaughtering the animals, some of their cells are harvested and grown outside of the animal in culture systems. Cells are fed with culture media and grown in bioreactors, large vats similar to those beer ferments in. This meat is then used in recipes for sausages or hamburgers like regular ground meat. Structured meats are produced by growing the cells on 3D structures, called "scaffolds," to guide cell growth and organization.
Most cells naturally possess the ability to divide and create new cells. They can do this to grow their home body, replace old cells, or heal wounded tissues. Indeed, in 1855 it was discovered that every cell in the world stemmed from another cell.
For many years, researchers have been looking for methods to grow cells outside a living organism. The first time cells were successfully grown outside a body was in 1907 using frog embryo nerve fibers. In 1916 new culturing methods improved the yield and quality of cells grown separate from an organism.
Instructing the cells to grow into edible muscle outside of a living organism requires specialized techniques and raw materials, both food safe and sustainable - plus a lot of trial and error. This process can roughly be broken down into four major steps:
- cell isolation, selection and growth
- structuring
- muscle formation
- post-culture and finishing
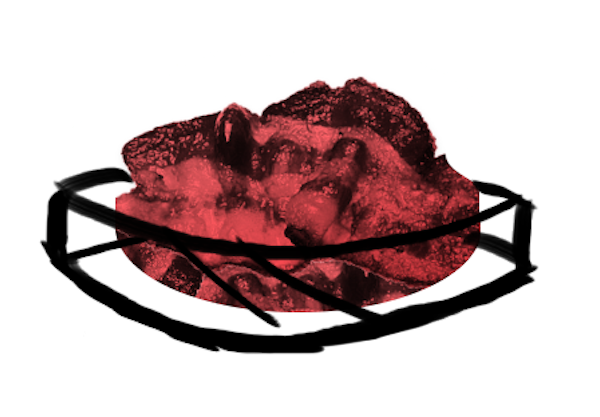
Obtaining the cells
Muscle tissue is composed of several types of cells, proteins, fats, polysaccharides and water. The production of cultured meat starts by obtaining some of those cells, including muscle fibers and stem cells. A small and pain-free biopsy with local anesthesia is performed on a living animal. This small piece of tissue is cut into even smaller pieces and digested with enzymes, similar to how food is digested in your stomach, to break the tissue into individual cells.
Researchers are still refining which cell types to grow and include in cultured meat. Not all types present in muscle might be needed. There are two reasons that make this not so straightforward: i) cell plasticity and differentiation potential; and ii) meat formulation being made.
Many kinds of cells such as nerve cells, endothelial cells (from inside blood vessels), myocytes (the cells that make up muscle fibers), adipocytes (fat cells), fibroblasts (cells from within the muscle), tenocytes (from the tendon), and stem cells are present in meat, and the necessity for each must be weighed like ingredients in a recipe. Each type contributes differently to the nutrition and texture of the meat. One might reasonably expect at least myocytes to be included to provide the muscle-y, fiber-like texture that is characteristic of meat, along with adipocytes to add the flavor, texture, and juiciness of fat.
Adult somatic cells are cells obtained directly from a biopsy. They are differentiated, which means they are more or less committed to their fate. So, if I sampled a muscle cell and grew it in my lab, it will produce more muscle cells and not, say, liver cells. These cells already display many of the characteristics of the muscle itself. However, to obtain pure adult somatic cells for each type listed above to grow in the lab may be an exhaustive and expensive process. Moreover, adult somatic cells grow slowly and are finicky to maintain.
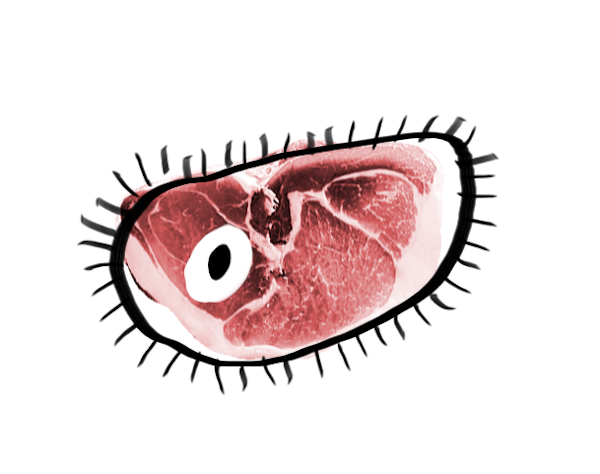
Stem cells are cells whose identity is not yet established and have the potential to differentiate into specialized cells. They can become your adult somatic cells, your adipocytes and myoblasts and whatever else is needed, and can be a better option for growing cultured meat than adult somatic cells. There are also different kinds of stem cells that are distinguished by their potency and source. The more "potent" a stem cell is, the greater number of different cells it can turn into. For example, a newly fertilized zygote is "totipotent:"it can turn into any other kind of cell in the body. The stem cells I grow in my lab are myosattelite (from within the muscle), adipose derived stem cells (from within the fat) and dedifferentiated adipose tissue cells (from the fat). While myosattelite cells are committed to being myogenic cells (they will only turn into and produce muscle cells), the others can differentiate into multiple types of cells and are more abundantly collected. This cell yield is an important factor to minimize the number and size of biopsies done. The yield and health of stem cells -- they grow faster and will produce for longer -- make them a better option over adult somatic cells. But, the specific kinds of stem cells that are best for different applications is still being worked out.
Cultivating the cells: bioreactors and culture media
To industrialize cultured meat huge cell production capability is necessary, far beyond that that an academic lab can achieve. That can be achieved with large bioreactors, essentially huge vats filled with a nutrient rich liquid called culture media. The culture media is what feeds the cells with salts, vitamins, proteins, and sugars that are necessary to grow.
The projected numbers are exciting: in just 2 months, 50,000 tons of pork cells could be grown per bioreactor, by using starter cells from 10 pigs. Estimates show that those ten pigs could provide about 0.8 tons of meat if they had been slaughtered. Another estimate suggested that one continuously running 25,000L bioreactor could provide meat for approximately 10,000 people.
There are different types of bioreactors but the most common are vessel or tank-shaped. They can look like a beer fermentor. Cells are immersed in culture media and kept under constant agitation to avoid sedimentation (pilling up at the bottom because they don't swim). Simultaneously, conditions like pH, CO2 and O2 content, and agitation speed are monitored. The cells may self-assemble into small aggregates or be attached to small biodegradable particles called cell micro-carriers that are added to the media. These allow the cells to maximize surface area, and thus space to grow, available to them.
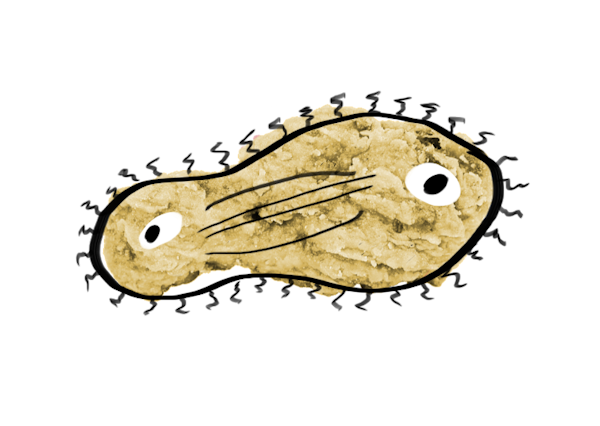
Since the first attempt to grow edible muscle, the composition of the media has been a point of major concern. One sticking point has been the use of fetal bovine serum (FBS). FBS is a mix of proteins, vitamins, and a variety of other factors that, right now, are essential for growing cells in vitro. It is often harvested from the blood of fetuses present in pregnant cows after they are slaughtered. Although FBS is considered a by-product of the beef industry, it is a major source of variability, not to mention safety and ethical concerns. To make industrial-scale cultured meat a reality, new media formulations that are FBS-free are needed.
About 800,000 liters of FBS are obtained annually from 2 million bovine fetuses. Again, FBS is not desirable but it is needed. It contains growth factors and many other compounds essential for cell survival, the sorts of things that cells would normally get from the body they inhabit. Therefore, producers are looking for alternatives like synthetic and chemically-defined sera (serum where every ingredient is precisely known and quantified), or media that is serum-free altogether, like using serum made from plant derivatives.
FBS also significantly increases the production cost. Half a liter of certified FBS can cost around $600 and usually represents 10% of the cultured media volume. To fill one 25,000L bioreactor, 2500 L of FBS would be needed. So, to feed 10,000 people every month with cultured meat, the FBS alone would cost $1,500,000.
Structuring the cells: scaffolds
The cultured meats hitting the market first will be unstructured meats. This means they will look like common processed meats, like ground beef, where the typical muscle fiber seen in things like steak or chicken is not apparent. To achieve the highly organised form of a steak or a chicken breast, the use of a structure to grow on is needed. Those three-dimensional structures are known as scaffolds. Imagine the 3-dimensional outline of a steak which cells grow on and fill in, like a newly built house. These scaffolds will be degraded by the cells during culturing, or removed. They can also simply be eaten in some cases. Example of edible scaffolds are made from plant leaves or alginate derived from brown algae, mushrooms, or other foods.
Not every type of possible scaffold may be immediately suitable because the ways the cells interact with them have to be optimized. Cell survival and behavior are dependent on their interaction with the surroundings, in much the same way cells within you interact with the rest of your body - but in this case it's the scaffold and/or the medium they are growing in. It is by interacting with the surrounding that signals (chemical, electric, mechanical) are naturally sent into the cells so they know what to do -- how to grow and proliferate. Cultured meat engineers are looking for scaffolds that are both edible, or removable, and allow for these signals to be transmitted.
There are two methods to structuring cells using scaffolds. The scaffolds can be previously prepared and cells added on to them. Alternatively, cells can be mixed with the raw ingredients for a scaffold, and the two allowed to build the meat together. In the first case, cells are seeded onto the sterile scaffolds. In the second, very small units made of scaffold and cells are assembled and positioned in a controlled manner. For example, a 3D bioprinter or a 3D food printer, dispenses a gel-like mixture containing a scaffold/cell mixture or only cells, layer-by-layer, following a computer assisted design. Imagine a high-tech pastry-bag. With this technology the type and content of cells could potentially be tuned at the muscle fiber level.
Muscle genesis
After the house is built, it is time for the cells do their job: become the muscle, become the fat, or become something else, depending on the meat recipe. This is the period where the scaffold with cells growing on it maintains conditions mimicking an animal body. Nourishing the cells with nutrients, bioactive proteins, and exercise will let them known that it is time to become muscle. This step of muscle tissue formation and maturation is known as myogenesis. The stem cells are differentiated into myoblasts, for instance, by delivering them the right bioactive growth factors, biological signals that direct stem cells to start becoming muscle cells. The myoblasts then naturally fuse together into long fibers called myocytes by reducing the presence of certain protein - e.g., "mitogenic growth factors" that would instruct them to grow and divide instead of forming muscle.

Simultaneously providing biological, mechanical, and electrical cues is important for closely mimicking the environment of an animal body, and for improving muscle formation or maturation. However, the timing and characteristics of those stimuli is still being studied. For instance, there is a consensus that mechanical stretching promotes myoblasts growth, but it also may inhibit muscle maturation. On the other hand, electrical stimuli can promote muscle fibers maturation. Electric cell stimulation is not weird as it might sound. Functional electric stimulation is used for human injury rehabilitation , and cell cultures can be electrically stimulated or "exercised" to promote cultured muscle growth and maturation in a similar way.
Finishing
A last step might involve processing or finishing the cultured meat so that it is ready to consume. As an example, the meats may be processed to create unstructured cultured meat products (e.g. chorizo, sausage, nuggets, burgers).
But, there are still many unknowns about how cultured meat will behave after being taken out of culture. Will post mortem rigor formation happen? If so, what are the best conditions to store the meat before consumption? Is the scaffold cohesive and robust enough to support storage and transportation? How can this meat be cooked? Many question have yet to be answered.
What will cultured meat look and taste like?
The first attempts at growing meat in a lab produced a less-than-optimal flavor and texture experience. However, there have been great strides made in the aesthetics of cultured meat in the past years.
Wheat-based seitan chicken wings and soy chorizo sausages are easily available at almost any large supermarket. The Impossible burger is the talk of the town in and out of the world of food tech startups. So are we already living in the futuristic world of meatless meat? Well, not really.
While companies producing plant-based meat substitutes are growing quickly, the future of cultured meat is still hazy. The first hamburger made entirely of cultured meat hit the grill in 2013, but the cellular agriculture industry is still facing many of the same challenges and setbacks from those early days.
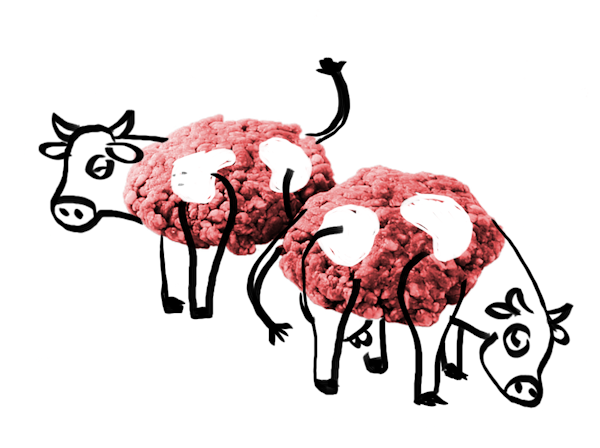
In order to be universally accepted by consumers, meat alternatives have to not just look like conventional meat, but also mimic its smell, texture and taste — not a trivial task for food scientists. A blend of over a thousand water soluble and fat-derived components make up specific flavors of different strains of meat, so accurately emulating the distinctive taste of meat is a difficult endeavor.
In 2013, both the taste and price of a cultured burger left a great deal to be desired. Not only was production cost $330,000 per patty, the texture of cultured meat was pretty much the opposite of conventional meat. Instead of being juicy and soft, it resembled a dense protein cake. However, it only took 2 years for the price to go down from $330,000 to $11.36 per patty or $80 per kilogram, and become at least marginally comparable to that of beef ($4.22 per kg). As the price improved, the taste did too. Paul Shapiro, author of a book on cultured meat, got to taste cultured foie gras made by California-based company JUST (formerly known as Hampton Creek). “The pâté was rich, buttery, savory, and very decadent, just as one would expect”, he wrote.
It turns out that making cultured foie gras is also easier than growing a burger, in part because it takes less effort to grow liver cells in culture than in a bird (ducks and geese are force-fed to induce hepatic lipidosis, or fatty liver). When cultured liver cells receive excess sugar, they begin to produce more lipids and get fattier and fattier, mimicking the texture of foie gras while eliminating the need for waterfowl cruelty.
Currently, one of the biggest challenges in cultured meat production is decreasing the cost of molecules that are essential for sustaining cell growth in culture. But because foie gras is already marketed as a luxury product with a cost of at least $50 per pound, getting a version from the lab to be cost-competitive is less difficult than bringing down the cost of cultured burgers.
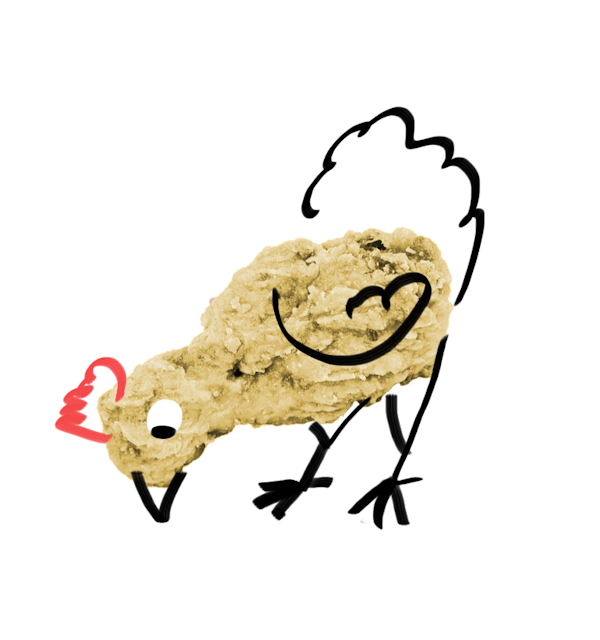
That said, it looks like cultured chicken wings won't be on shelves any time soon. Unlike chorizo, foie gras, or even a burger, whole-muscle meat requires a structure (or scaffold) and constant supply of nutrients to its center; in other words, growing a pork chop is much more complicated than growing a hotdog. Scientists say that they are still very far away from growing steaks in lab and even further from achieving the same flavor quality as whole-muscle meat from living animals.
No matter what cultured meat tastes like, manufacturers will have to overcome buyers’ concerns about safety. In a consumer behavior study, people who were apprehensive about eating cultured meat were primarily worried about potential health risks down the road. But those fears appear to be unfounded: in principle, cultured meat should be safer than conventional meat. The chance of microbial contamination is much lower under strictly-regulated lab conditions. Cultured meat will be less exposed to bacteria and fungi that can spoil the product and cause food-borne illnesses. Because of how much control scientists have over the production process, it is conceivable that cultured meat could contain less saturated fat and more beneficial fatty acids. In a similar vein, antibiotics and growth-promoting hormones routinely given to livestock for traditional meat don't have to be present in cultured meat. However, nitrates, potentially carcinogenic food preservatives, will still be present to ensure an adequate shelf life.
With so many cultured meat producers working to decrease costs, make their meat taste more familiar, and win over consumers, it's possible that cultured meat will soon be as easy to buy as tofu or seitan.