Chapter 1
The organisms we modify
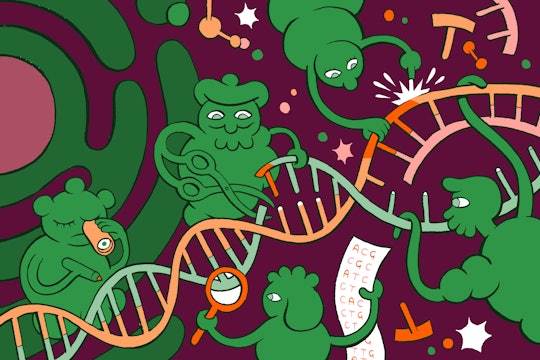
Matteo Farinella
What is genetic modification, generally?
The term “genetic modification,” sometimes called "genetic engineering," typically refers to when DNA is modified directly, on the molecular level. But that definition is pretty slippery.
In the 1970s, the US was staring down the barrel of an insulin shortage. Diabetes, once a virtual death sentence, could be easily controlled with injections of insulin derived from the pancreas glands of pigs and cattle. But making just one pound of pure insulin required over 20,000 animals, and as the demand for insulin rose, manufacturers worried that they wouldn’t be able to keep up.
The solution seemed like something straight out of science fiction. Researchers at a fledgling company, Genentech, inserted the genes for human insulin into a harmless version of the bacteria E. coli, transforming the bacteria into tiny, insulin-producing machines. These bacteria could be grown in massive fermenters, like the ones used to brew beer, churning out nearly unlimited quantities of the drug. The bacteria-derived insulin, named humulin, was approved by the Food and Drug Administration in 1982, making it the first product on the market to be produced through genetic modification.
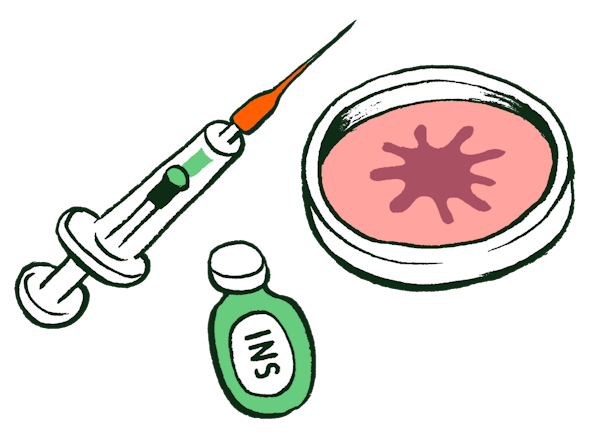
So how did the researchers coax bacteria into making a protein from an entirely different species? The first step was to build strands of DNA containing the instructions for making human insulin.
Insulin, like all proteins, is composed of molecules called amino acids that are strung together to make a chain. You can imagine this like a necklace, where every bead is a different kind of amino acid. Which amino acids are part of the chain, and what order they’re in, is what gives the protein its unique function.
The process of making a protein starts with DNA. DNA is also a strand of smaller molecules, each of which contains one of four chemicals called bases: adenine (A), thymine (T), guanine (G), or cytosine (C). The instructions for making a protein are encoded in the sequence of bases.
The process is a little different in bacteria, but the basic order of DNA to RNA to protein is the same
The sequence of DNA that contains the code for making a particular protein is called a gene. To make the genes for insulin, the researchers used a method called gene synthesis, a genetic engineering tool still used today. They took small pieces of DNA and, through a series of chemical reactions, strung them together in the right order.
The next step was to insert the gene into a small circle of DNA, called a plasmid, so that it could be taken up by the bacteria. First, they cut the plasmid using restriction enzymes. Restriction enzymes are often portrayed as molecular scissors because they cut chemical bonds in DNA. But they don’t cut DNA just anywhere. Each type of restriction enzyme recognizes a specific sequence of DNA and only cuts there, allowing researchers to cut DNA at a specific location.
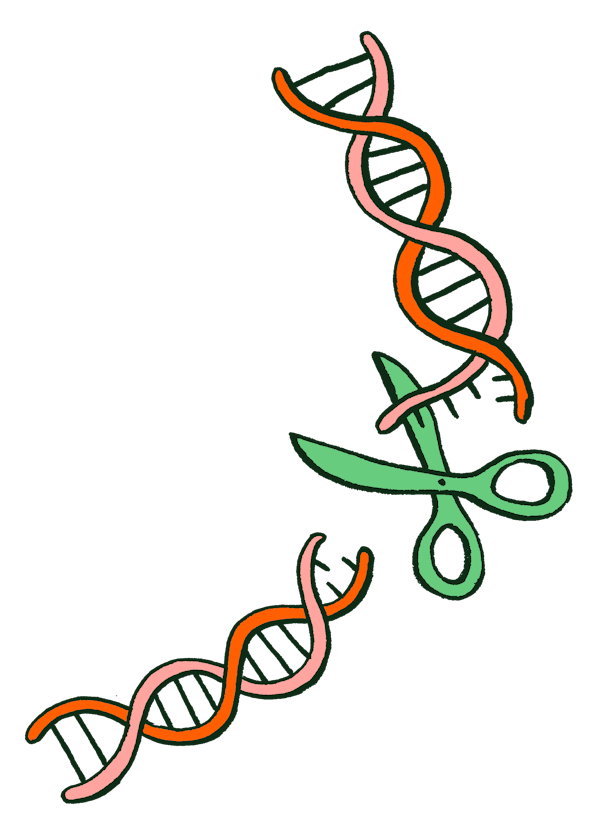
Next, they added their synthetic gene and used a protein called DNA ligase to stick it all together. Using restriction enzymes and DNA ligase to link different pieces of DNA together is the first kind of recombinant DNA technology. This method, called “cut-and-paste cloning,” is also still used today.
More recently, a new approach called CRISPR has made genetic modification much easier. In this approach, a piece of RNA guides a protein called Cas9 to the region of DNA to be modified. Cas9 then cuts the DNA in that location. When the cell repairs its DNA, it fills in the break by copying from a strand of DNA that the researcher provides. By providing different template sequences, it's possible to delete, insert, or change the sequence of almost any gene.
The term “genetic modification,” sometimes called "genetic engineering," typically refers to when DNA is modified directly, on the molecular level. But that definition is pretty slippery. Since the early 20th century, people have produced new varieties of plants by exposing seeds to radiation or to certain chemicals called mutagens. This damages the seed's DNA, and when the plant repairs the damage, it sometimes adds the wrong base, changing the DNA sequence. Although this process directly changes DNA, resulting in plants that do not occur in nature, it is also not considered a GM technique by regulatory agencies, because we were doing it since before the advent of modern genetics.
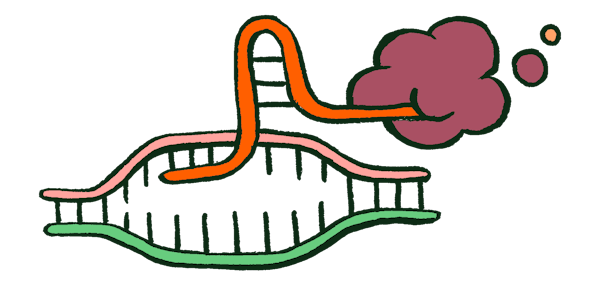
It's surprisingly difficult to pin down a conclusive definition of "genetic modification." That means that when deciding whether a product is healthy or unhealthy, dangerous or unsafe, it's not enough to know whether or not it's genetically modified. The important part is exactly what genetic change was made, no matter what tools were used to make it.
What is GM of crops, why do we do it, and how is it done?
Genetic engineering is the process of changing an organism's genome to give it a desirable characteristic, and there are a handful of GM methods originating from research done in the 1970s that we use today. There are many reasons to engineer crops: to suit tastes, increase yield, tolerate rapidly changing environmental conditions, resist pests, or create medications.
A species' collection of traits doesn't usually change very quickly. The hair on your head, walking upright, and even color vision, evolved gradually from the fur, feet, and eyes of humanity's ancestors. Numbers of teeth, two legs or four, the pattern of flowers, the sweetness of fruit – they all evolve slowly.
In the distant past, if you were a corn farmer, you planted a very small relative of the modern, sunny yellow variety. If you wanted your plants to, say, grow taller, your options were limited. You could do nothing and hope life worked out OK. Or, if you were more proactive and also lucky, you might find one plant in your whole field that grew a smidge taller. Not a lot – just a touch. Being better than nothing, you start exclusively planting that slightly-larger-than-average plant. This process continues, with the slightly taller plants getting selected and cultivated, year after year, until there are fields of tall green stalks, weighed down with heavy yellow ears, all over the countryside.
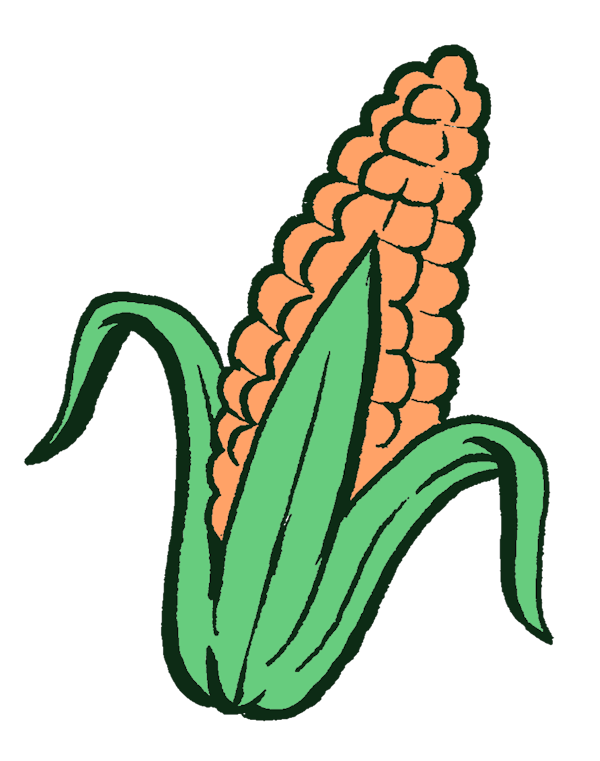
This process of selecting and breeding the most desirable corn is crop engineering. Unwitting weavers of a genetic loom, every selection for larger kernels, sweeter taste, or greater yield changes the corn genome. The process is often slow (though it's still a useful technique today), and the selection of traits is usually small and barely distinguishable from one another. Changing corn from the small, ancient variety to the large modern one took 5,000 years. But nevertheless, this picking and choosing of valuable attributes is not so different from modern genetic engineering.
In the past, these selections were made the old-fashioned way: plant sex. Sexual reproduction gives each offspring plant half of their genome from one parent and half from another. Mating two plants that individually have the traits you want – maybe one has a huge yield but doesn't grow well on your plot of land, while another barely produces crop at all but grows like gangbusters – is a convenient way to hopefully produce plants that have the best features of both.
This leaves you with a limited selection of traits, though. Anything that can't be bred into a crop from the same species or a related one is off the table. That would leave you with no recourse but waiting for new traits to evolve. But, if you could artificially insert the gene for a trait you wanted, then the list of possible traits becomes potentially endless.
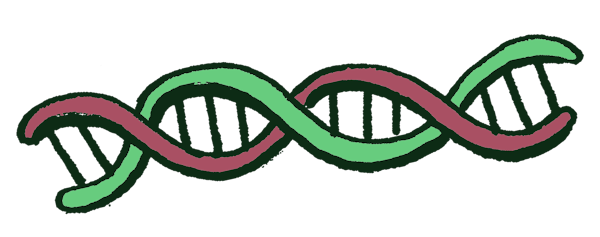
Whether slowly, generation by generation, or quickly in a modern lab, genetic engineering is the process of changing an organism's genome to give it a desirable characteristic. There are many reasons to engineer crops: to suit tastes, increase yield, tolerate rapidly changing environmental conditions, resist pests, or create medications.
There's also another advantage. It's counter-intuitive, but once you notice, it's hard to forget: engineering a crop specifically for the trait you want actually involves a much smaller change to the genome than breeding. When you breed crops in pursuit of specific traits, as it's been done for millennia, you make wholesale changes to the plant's genome. If you examined each variation between two related plants, their differences at the genetic level would number in the thousands or tens of thousands. Taking two plants, making parents of them and mixing their genomes 50/50 causes a huge, wide-scale change. In trying to acquire one feature of a plant, you could bring in other, unwanted traits or leave another out. Making one, targeted, specific change preserves far more of the original genome.
Many different modifications have been made in a number of different crops, but there are two common goals: reducing insecticide use and conferring herbicide resistance. One of these modifications adds toxic proteins to the plant's genome that targets Lepidoptera, caterpillars and butterflies, that eat and damage crops. The proteins come from Bacillus thuringiensis, a common soil-dwelling bacteria. Any crop with these proteins often gets nicknamed "Bt."
Bt crops are safe to eat for humans, other mammals, and even other insects, because the toxic proteins are only activated in the stomachs of Lepidoptera when they nibble on a plant. A pest, like the European corn borer, chews on a Bt corn ear, for instance, and dies, while a human can walk up to the same ear of corn, slather butter on it, and chow down with no detrimental effects. A farmer growing Bt crops doesn't need to use as much spray-on insecticide, which can have broad effects on other insects and the environment, since their crops produce their own, highly specific insect resistance. In a 10-year period, use of Bt modifications in just two crops, corn and cotton, resulted in a 30 percent worldwide reduction in insecticide use.
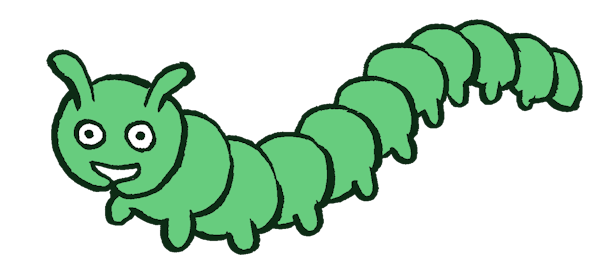
The other common modification gives crops tolerance to glyphosate, commonly known as RoundUp, the commercial name given to it by Monsanto. Glyphosate targets a protein, called EPSP synthase, involved in creating amino acids, the individual units of proteins. If EPSP is inhibited by glyphosate, the production of other proteins is inhibited and the plant dies. Glyphosate-tolerant plants carry a modified version of EPSP that is unaffected by glyphosate (so they're "RoundUp Ready"). So, a farmer can spray a field with the herbicide, weeds that compete with and crowd out crops die, and the crop lives.
Engineering Crops: How?
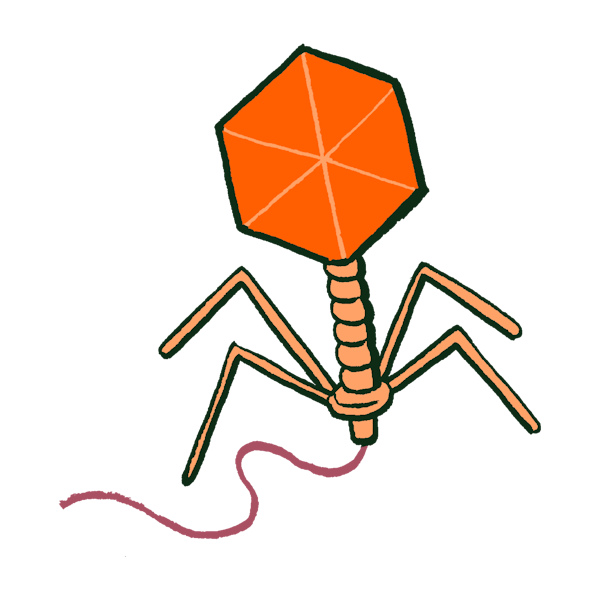
Biologists never miss an opportunity to turn a discovery into a tool. In the late 1970s, when scientists were studying bacteria that can cause tumors on plants, they saw these tumors forming because the bacteria had inserted a piece of its DNA directly into the plant's genome. This caused a mutation and cancerous growth, much like how cancers can form in humans. In less than five years, that bacteria, Agrobacterium, was used as a delivery service in plants for whatever DNA sequences scientists wanted. They could alter the bacterial genome easily, cut out the cancer-causing DNA, replace it with their own desired genes, and that DNA would end up in the plant's genome instead. This can be used for simply delivering one gene to a random location in the genome (though it can also be used to deliver genes for CRISPR proteins, which can make more precise changes).
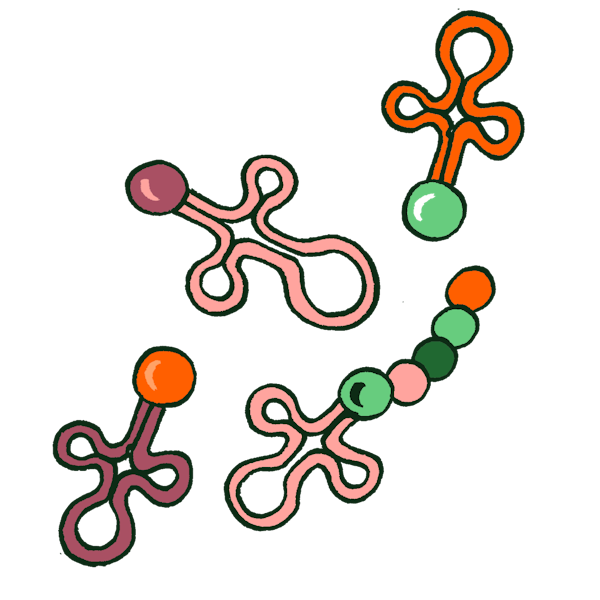
That's one approach to getting new DNA into a plant. But there are many more, each with their own technical advantages and limitations – fascinating differences, if you're the kind of person who talks about technical advantages and limitations at parties.
There's another approach worth mentioning: biolistics. If that sounds like a word for a gun that shoots genes, your ears do not deceive you: colloquially, it's called the gene gun. It's a device that turns DNA into a projectile, literally propelling the gene coding for a desirable trait at high speeds so it penetrates a plant's exterior. It's a useful and popular way to deliver DNA to plants, and follows the amusing logic of "have we tried just shooting the plants?". Similar to Agrobacterium, gene guns can be used to deliver genes directly, or you can shoot CRISPR proteins for more elegant, precise changes. Other methods, like electrically shocking plants in a way that opens pores in plant cells' membranes, allowing DNA to be sucked in, are less commonly used to make GE crops (though in research settings it is one of the more common ways plant biologists use CRISPR in the lab).
How common are GM genes in our crops?
Corn and soybean alone account for 93 percent of all GM crops in the US (each is 46-47 percent of the GM crop market). Because corn and soy byproducts are ubiquitous in most processed foods, and ‘living modified organism"’ plants (like a tomato or beet) are much harder to find, your best bet in avoiding GM crops is to avoid processed foods that contain soybean or corn or their byproducts.
All the crops in the United States cover about 318 million acres, an area about twice the size of Texas. A 2016 estimate put the acreage of GE crops at 180 million, or about one Texas. In other words, roughly 57 percent of all crops planted in the US are genetically engineered in some way.
But if you account for the most common GM crops, those numbers shrink dramatically. Corn and soybean alone account for 93 percent of all GM crops in the US (each is 46-47 percent of the GM crop market). If you add cotton, which most people don't eat, that accounts for 99.81 percent of all GM crops in the country. All other GM crops grown in the US – squash, potatoes, apples, among others – is in that leftover 0.19 percent.
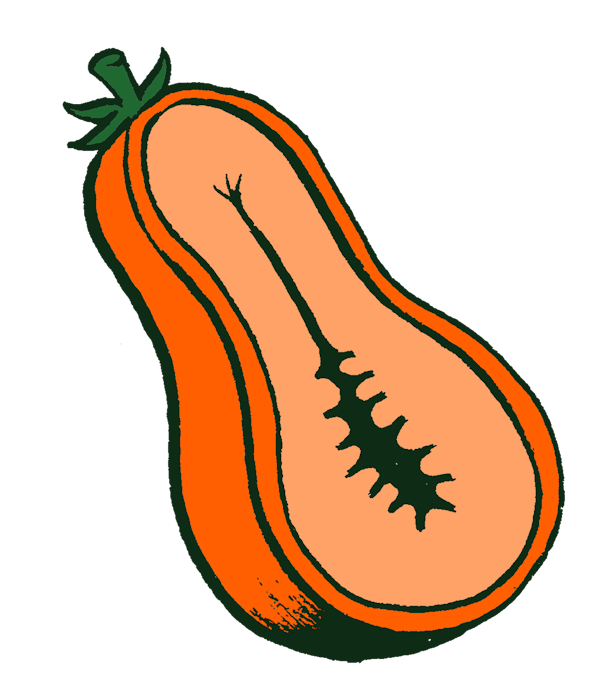
Most corn grown today is either glyphosate-resistant, Bt-modified, or both (crops that carry both are called "stacked"). Corn is everywhere – corn starch, corn syrup, corn oil, processed corn meals, and animal feed, for starters. It's useful and ubiquitous. Add to that the nearly complete takeover of the soybean market by engineered plants, avoiding GM crops in some way would be nearly impossible – especially since soybean products are also in everything, from soy sauce to soybean oil to biofuels, from paint stripper to AstroTurf. Anything that contains corn or soy as an ingredient could contain a GM ingredient. Estimates vary, but most put 70 to 80 percent of processed foods in the US as having some kind of modified ingredient.
Finding an actual, whole GM crop (the precise phrase is "living modified organism") in your supermarket is much more difficult. There just aren't that many of them out there. This is chiefly thanks to the difficult regulatory process for getting one approved for sale in most places.
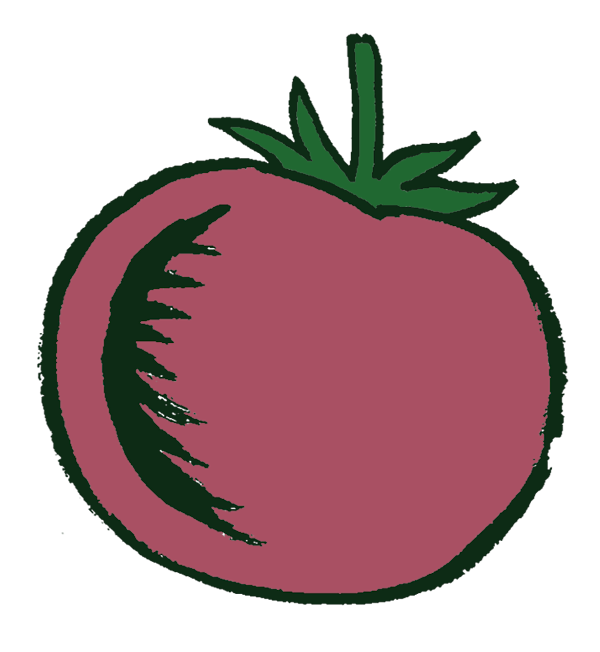
But there are other reasons. The first whole GM food available was the Flavr Savr tomato, which ripened more slowly than other tomatoes to allow it to stay on the vine and remain fresh longer. It was on the market starting in 1994 but was soon removed from stores because it tasted terrible. But, other GE vegetables are available today, like virus-resistant squash, which come with macho, flag-rippling names like Freedom II and Liberator III.
Until 2017, the only whole, actual engineered fruit available in the US was papaya. Papaya in the US is mostly grown in Hawaii, where it was first introduced in the 1910s from Barbados and Jamaica. Commercial harvest began in the 1940s on the island of Oahu. Soon after, farmers discovered the crop was infected with papaya ringspot virus, spread by aphids and rendering the plant stunted and misshapen. Infected crops yield fewer fruit, and the fruit that is left behind tastes and smells bad. In the 1950s, papaya harvesting was moved to the Big Island, where the virus didn't yet exist. But the virus slowly encroached over the decades, even when, In 1978, research into controlling the virus began. By the late 1990s, the Hawaiian papaya industry was on the brink of collapse. In 1997, the FDA and EPA gave approval to virus-resistant, GE papaya. Within three years, most Big Island papaya growers were planting GE crops. Now, all papaya grown in the US is a GE variety resistant to the ringspot virus, though non-GE papaya is still grown in other parts of the world.
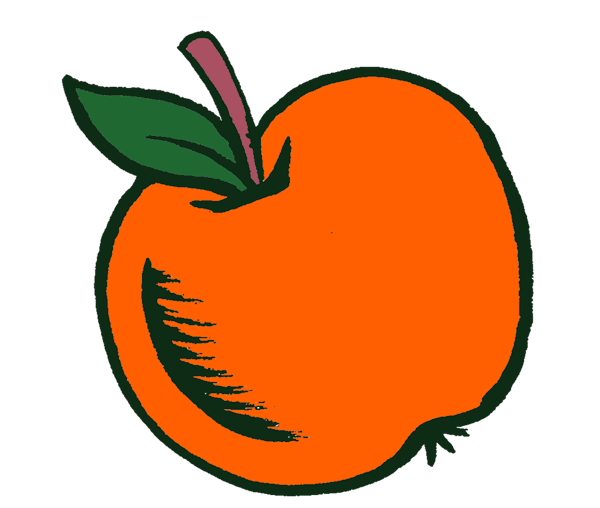
Recently, the Arctic apple was introduced to the US market. This apple's distinction is that it doesn't turn brown after being cut. While that doesn't sound like much of a change, proponents argue this change will cut down on food waste, since people won't be inclined to throw out a discolored but otherwise edible apple. It's also unique because instead of inserting a gene from another species, this apple is modified to produce less of an existing gene, the one responsible for browning; its modification is entirely within the apple's own genome.
The list of GE food available is not lengthy but if you enjoy databases, feel free to peruse one that sorts by plant, trait, and country here. They include alfalfa engineered to have less lignin and make more digestible animal feed, virus resistant plums, and carnations engineered to be colored blue.
Where are GM crops being eaten?
GM crops are eaten all over the world. But attitudes toward them – and regulations – vary from country to country, to the point where it's hard to keep track of who's doing what, and why. In total, 28 countries are planting GM crops, on every continent except Antarctica.
GM crops are eaten all over the world. But attitudes toward them – and regulations – vary from country to country, to the point where it's hard to keep track of who's doing what, and why. In total, 28 countries are planting GM crops, on every continent except Antarctica. The United States and Brazil lead these nations in terms of production, followed by India, Bangladesh, China, Pakistan, South Africa, Sudan, Burkina Faso, Cuba, and Australia, among others.
There is no federal legislation in the US regulating the growth or importation of GM crops. In the EU, only five countries grow them: Spain, Portugal, Romania, the Czech Republic, and Slovakia. But even the countries in the EU that don't grow GM crops still import them. In the rest of the world, another 31 countries allow GM crops imports, with 59 countries, in all, where GM crops are available, including everything from cotton, potatoes, corn, soybean, to canola.
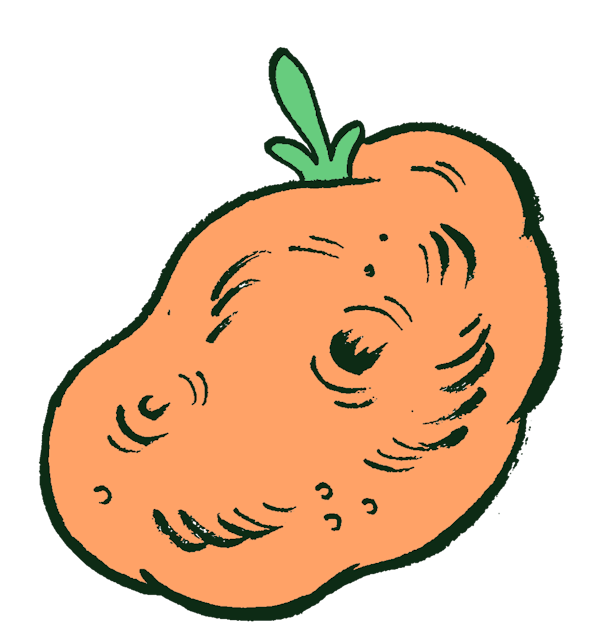
On the other hand, there are about as many countries that explicitly ban the growth or importation of GM crops. The largest country with a ban is Russia, which recently signed a law banning GM crop cultivation, although regulated imports are still allowed. Most of the EU also bans their growth, although attitudes vary from country to country. In some parts of Africa, attitudes can be similarly hesitant. Several countries, like Benin and Zambia, among others, ban GM crops, although a number are loosening restrictions. Kenya, Uganda, and eSwatini (formerly Swaziland) are all conducting field trials with GM crops.
Reluctance to allow GM crops comes from a number of concerns. Many folks fear that engineered genes will spread into the wild or that GE crops might have adverse effects on human health. In some developing nations, in addition to human health or environmental fears, leaders fear that giving power to foreign biotech companies could turn political tides. Acceptance of pest-resistant corn into India was delayed in part for this reason. In October 2017, the Ugandan parliament passed a law allowing GM crops, but it wasn't signed by the president, in part over concerns of corporate colonialism, and also from human health and environmental concerns. The bill was re-introduced in late April 2018 with language that could imprison farmers or scientists if any adverse environmental effects of GM crops (whatever those might be) were discovered.
How are GM crops regulated?
There isn’t a simple answer. There is no federal legislation in the US regulating the growth or importation of GM crops. The regulation of GM crops in the US is messy and involves three agencies: the EPA, the FDA and the USDA.
The regulation of GM crops in the US is a complete mess. Three different agencies have different areas of oversight, depending on the crop, the modification, and what purpose it was made for; you can see a summary here. Again, the growth or importation of GM crops is not regulated. What is regulated is the sale and consumption of those crops as food, so you can grow whatever you want as long as you don't sell it.
The Food and Drug Administration (FDA) is responsible for all food safety issues in the country. With GM crops, they pay attention to things like changes to the nutritional content (that table on the side of a cereal box) or the potential inclusion of an allergen into a crop.
The Environmental Protection Agency (EPA) examines environmental concerns, with particular interest in pesticides and the spread of pesticide-related products to the environment. That can include things like "Bt" crops, which carry genes that give them resistance to insect predators, all the way down to tiny pieces of DNA that fine-tune expression of other proteins.
Finally, the United States Department of Agriculture (USDA) examines the safety of planting GM crops and field testing them for approval.
If some of those roles seem like they might overlap, it's because they do. Some crops require different combinations of review from different agencies. The recently released Arctic apple, which does not brown after being cut, was looked at by the USDA and the FDA, but not the EPA. Bt corn, however, was examined by all three agencies. Approval is given on a case-by-case basis, needs to be OK'd by any agency that claims oversight, and requires hundreds, if not thousands, of pages of documentation.
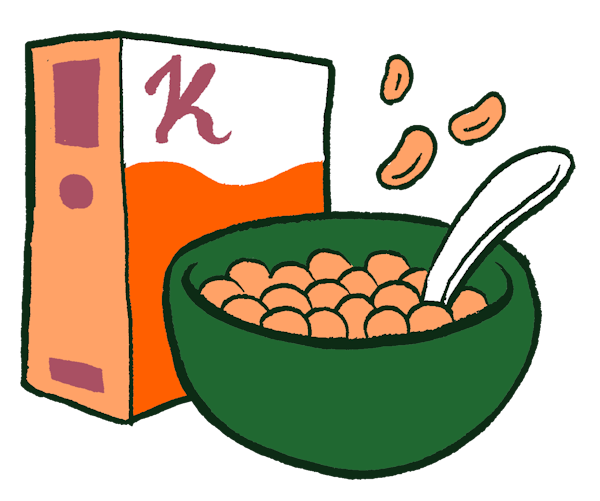
Other parts of the world take their own approaches, but for the most part it's an arduous process. In the EU, each crop is considered case by case, and only approved for 10-year periods. The marketing and import of GM crops is regulated by the European Commission, the legislative body of the EU. But each member of the EU is free to pass its own sub-regulations to restrict the sale or cultivation of GE crops within their own borders.
In addition to their own laws and regulations, 171 countries enforce the Cartagena Protocol, an international agreement to protect the environment from any potential risks that genetic modification might pose (the US is one of the few countries that doesn't enforce it). The Protocol does a lot of things: it requires exporters of GM crops to inform their importers that they're getting engineered crops, both for consumption and for release into the wild (like modified seeds or fish); it also establishes an online repository for information exchange about their engineered crops. What it doesn't do is require product labeling for consumers or speak to food safety issues in any way.
How are GM crops labeled?
In the US, there is no requirement to inform consumers whether a food is engineered or not. The FDA offers guidelines to anyone who wants to do so, but from their point of view it's voluntary. The USDA is currently taking comments until July 3, 2018 from the public on proposed labelling for ‘bioengineered’ products.
Labeling is a messy and complicated fight. Biotech companies that produce GM seeds and crops lobby against labeling, because they believe it diminishes their sales. Organic food companies lobby for them, to drive consumers to them. Advocates for labeling list several concerns that generally fall into two categories:
- Concerns for human health – from the direct product of engineering or from overuse of herbicides and pesticides on crops engineered to be resistant to them.
- And the simple right to know that a food they're eating is engineered (similar to labels that state that a food was produced in the same facility that nuts were processed).
Opponents fear that labeling might reinforce a stigma against GM food; that restructuring of infrastructure to separate non-GM and GM crops would raise prices (for example, should a farmer who plants both require two sets of equipment to the crops from fraternizing?); and that the economic (like higher yields increasing farmers' income) or environmental benefits of GM crops (like reduced water usage) would be lost if they go off the market. Interestingly, there's evidence that foods that are labeled "free from GMOs/pesticides" or something similar are seen as healthy, even if the label doesn't make health claims at all.
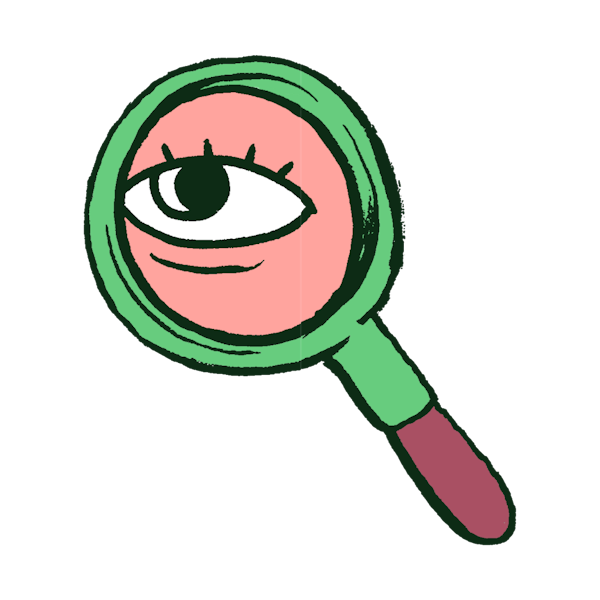
Deciding whether or not a food should be labeled as GM is tricky. For instance, does something highly processed like corn oil need to be labeled as engineered? If the oil is derived from, say, Bt corn, which carries proteins toxic to the insects that eat corn, those proteins (and the DNA that codes for them) won't be present in the final oil product. Is the resulting oil a GMO?
Questions like these, if not specifically addressed, can lead to situations like China's. Labeling GM crops is nominally mandatory there, but guidelines for what should or should not be labeled are vague (for instance, it's unclear if foods that contain ingredients from GM crops need to be labeled). The imprecise directives have made confusion and noncompliance widespread.
In the US, there is no requirement to inform consumers whether a food is engineered or not. The FDA offers guidelines to anyone who wants to do so, but from their point of view it's voluntary. In summer 2016, US Congress passed a law that directed the USDA to come up with federal labeling guidelines within two years. That law also specifically prevents states from creating their own labeling laws (and so will supersede laws in Connecticut, Maine, and Vermont that already require it).
On May 4th, the USDA released their proposed, though not finalized, labeling rules. They settled on using the term "bioengineered" as opposed to "genetically modified" to refer to food carrying genes inserted by humans. Any engineered food for human consumption (including things like chewing gum) would be labeled. Processed foods like oils that don't contain DNA or the product of an engineered gene (like the protective proteins Bt corn carries) would not need to be labeled. Processed foods that only contain engineered ingredients (like cornmeal) would be labeled. For the labels themselves, the guidelines offer a few options for labeling, including a short sentence ("Contains a bioengineered food ingredient"), a symbol on the label indicating an engineered food (similar to a symbol denoting kosher status) or a QR code. The rules are open to commentary and likely won't be finalized until the end of summer 2018.
In the EU, labeling is compulsory on packaged food for anything that contains more than 0.9 percent GM food or ingredient. For any whole food GM crops, it has to be indicated nearby (as in, near the food in the store) that the food is engineered. Other countries like Japan and Australia also require GM foods to be labeled. Only two African nations – South Africa and Kenya – require labeling, although Kenya has not approved any GM crops for sale yet.