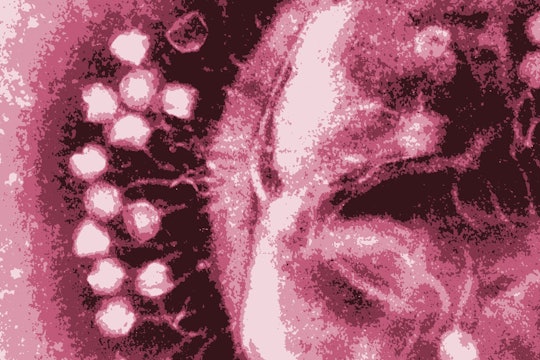
How CRISPR proteins work together to capture foreign DNA
A biologist writes the CRISPR explainer you've been looking for
Imagine a never-ending microbial war between bacteria and bacteria-eating viruses called phages. Phages are vicious bacteria killers, sinking their hooked legs into the bacterium and injecting their DNA, hijacking bacterial machinery for its own uses. One phage can multiply in minutes, bursting the zombiefied bacterium open, spewing out phages that can now infect more bacteria: outbreak!
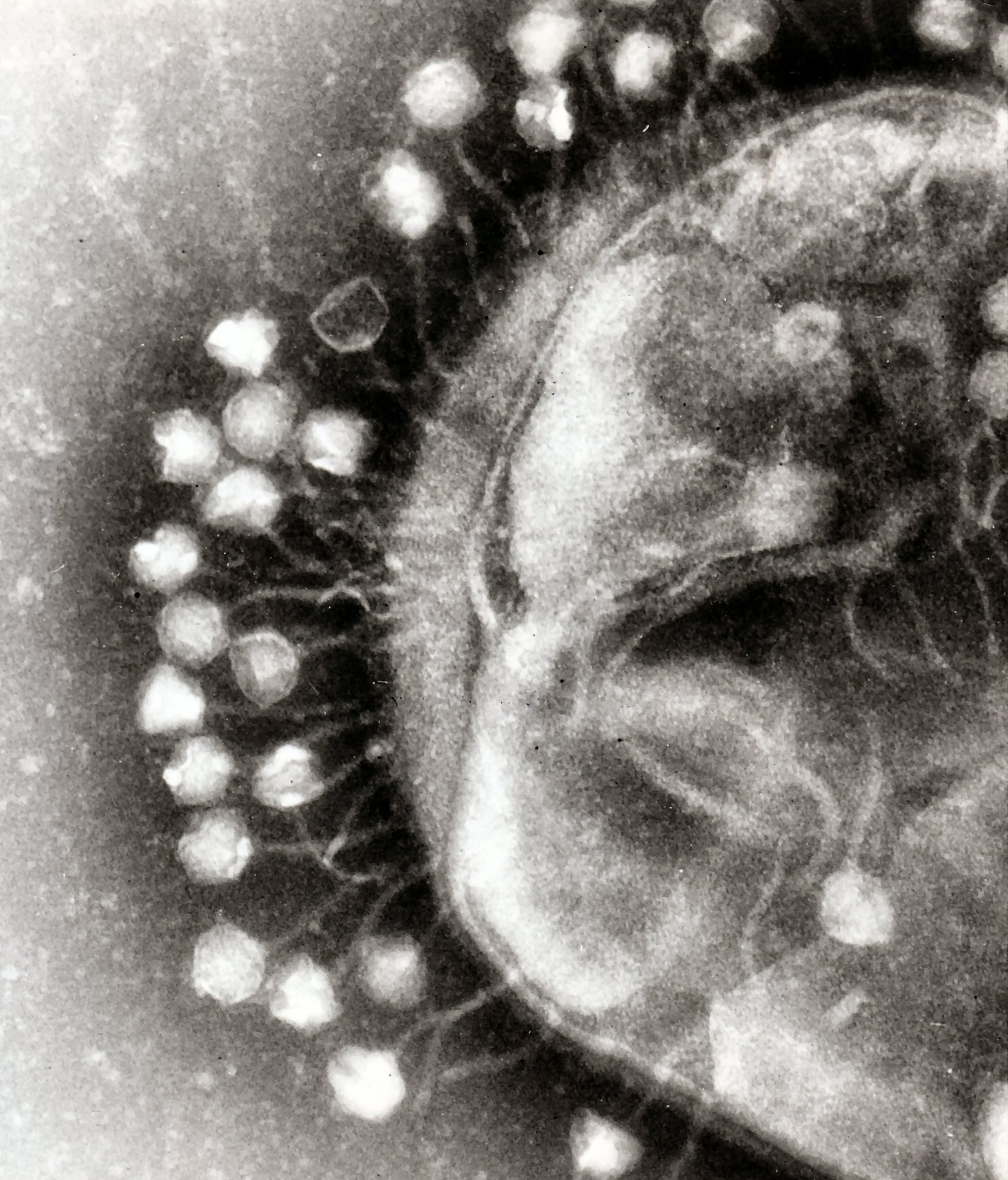
Electron micrograph image of phages attacking a bacteria cell
Bacteria, on their part, have evolved an adaptive immunity to attempt to combat phages: CRISPR. The term was in the news a lot recently when the world was obsessed with the GIF of an 1878 galloping horse stored in bacteria and again when presented with the possibility of 'designer babies.'
But our basic knowledge of how CRISPR actually works is still very limited, and the recent findings, ripe with premature projection, get far ahead of the fascinating, complicated process we are just beginning to understand. There are thousands of different CRISPR systems in nature, and we have only studied a handful of them, yet our limited discoveries have resulted in several breakthroughs.
Here is what we do know: CRISPR works by snipping off a piece of the virus and sticking it into a specific part of its own bacterial genome that then works as a mugshot, allowing the bacteria to recognize the virus if it tries to strike again.
For CRISPR to work properly, bacteria need a captor to capture a piece of the enemy, and an executioner to use the mug shots to target the virus thereafter. These jobs are done by proteins, which we call CRISPR Associated (Cas) proteins. You may have heard of Cas9, which is in fact an executioner. Bacteria did not evolve Cas9 so we could edit embryos; it evolved to fight phages. (As you can tell from the number, there are other executioners in the bacterial world, only one of which is Cas9). This is where the story of the horse GIF diverges: it does not use Cas9.
The process of grabbing a piece of virus to stick into the CRISPR mugshot collection is called adaptation. In all CRISPR systems studied to date, two Cas proteins, Cas1 and Cas2, form an alliance to capture a piece of DNA from the invader. Now imagine that instead of random phage pieces, you bombard the bacteria with 'mugshots' that can be decoded as a GIF of a horse. Cas1/Cas2 will stick that video into the CRISPR mugshot collection. That is exactly what Seth Shipman and colleagues at Harvard did in July, as a great example of how basic research on bacteria can turn into transformative technologies. But again, we are just starting to appreciate the complexity in play.
Here are some of the things we do know from a decade of research on CRISPR adaptation in E. coli: four Cas1s and two Cas2s come together in a six-piece complex, take a piece of the invader, and stick it into the CRISPR mugshot collection. We also know that a separate protein, called the integration host factor (IHF), acts like one of people holding orange batons at the airport, telling Cas1/Cas2 where to land. But we had never seen the entire complex in action, until a study, published in Science last month, took advantage of structural biology to capture the structure of the captors in action.
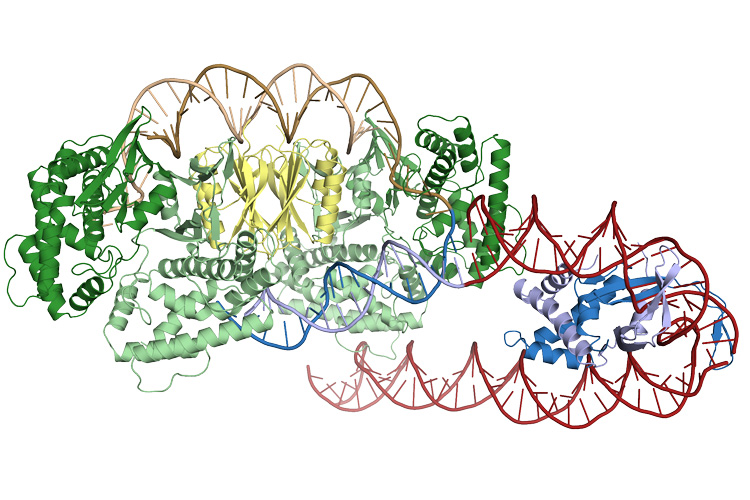
IHF (blue) creates a sharp bend in the DNA (red helix), which allows Cas1 and Cas2 (green and yellow) to recognize and bind the insertion site
Addison Wright and his colleagues at UC Berkeley show in that research that adaptation is far more interesting than we understood. The captor complex acts like a ruler, holding on to a snippet of a certain size, ready to be added to the collection next to the other mugshots. This is where IHF comes in; it holds onto that region of the bacterial genome, and bends it, which is like a secret message for Cas1/Cas2 to land there. As Cas1/Cas2 approaches, IHF holds on to Cas1 to ensure perfect landing, so that Cas1 can now integrate the new snippet into the mugshot collection.
I’d argue that the reason this is important goes far beyond our ability to record movies into bacteria. I mentioned previously that there are different executioners besides Cas9 (like Cas13a, Cas12), but Cas1/Cas2 seem to be the universal capturer proteins. They are fundamental to CRISPR working the way it does, and understanding them will reveal aspects of the microbial world that we never knew existed.
There are thousands of different CRISPR systems, some in our very own bodies. We're just starting to understand the intricacy of the microbial life going us all around us, and inside of us.