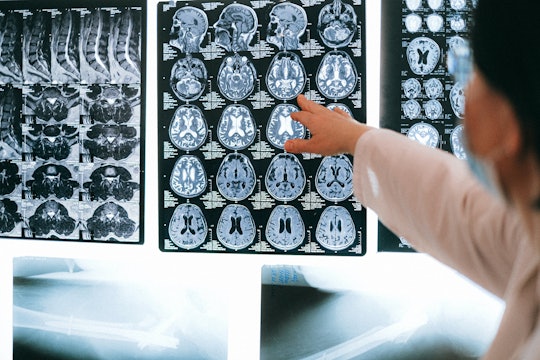
Anna Shvets via Pexels
A new molecule and an under-appreciated neuron have been implicated in Parkinson's disease
Researchers studying Parkinson’s disease pivot from the usual dopamine story and reveal a new mechanism underlying early motor deficits
In 1817, James Parkinson described the symptoms of his namesake disease in Essays on the Shaking Palsy. In his patients he saw movement abnormalities, including altered postures and tremors.
As the medical field expanded their understanding of Parkinson’s disease over the next 200 years, so have the available treatments, though there is still no cure despite it being the second most common neurodegenerative disorder. Perhaps invigorated by the stalls in new therapies available for brain-related diseases, researchers have started adopting new perspectives to study the brain. For Parkinson’s disease, this includes looking at more than one type of cell.
Decades after Parkinson’s symptoms were first vaguely attributed to the brain, physicians started speculating that damage to a particular brain region was the cause of the motor problems. This region of the brain is dark in appearance because of pigmented cells and is aptly named the “black substance” in Latin, or substantia nigra. As these cells die, there are less pigmented cells, making this brain region lighter in color. The pigmented cells normally release dopamine, and the loss of these cells is thought to underlie the many symptoms of Parkinson’s disease.
Cells talk with each other. For example, dopamine is a neurotransmitter famous for its association with happiness, but it's also critically important for movement. Parkinson’s disease is caused by the death of dopamine-releasing cells that project to and communicate with another brain region called the striatum. Dopamine is used as a language to communicate with medium-sized prickly-looking neurons called medium spiny neurons. These projecting neurons also disappear in people with Parkinson’s disease, so popular ideas for tackling Parkinson’s disease have been to restore dopamine availability in the striatum, such as by giving patients precursors of dopamine (L-DOPA).
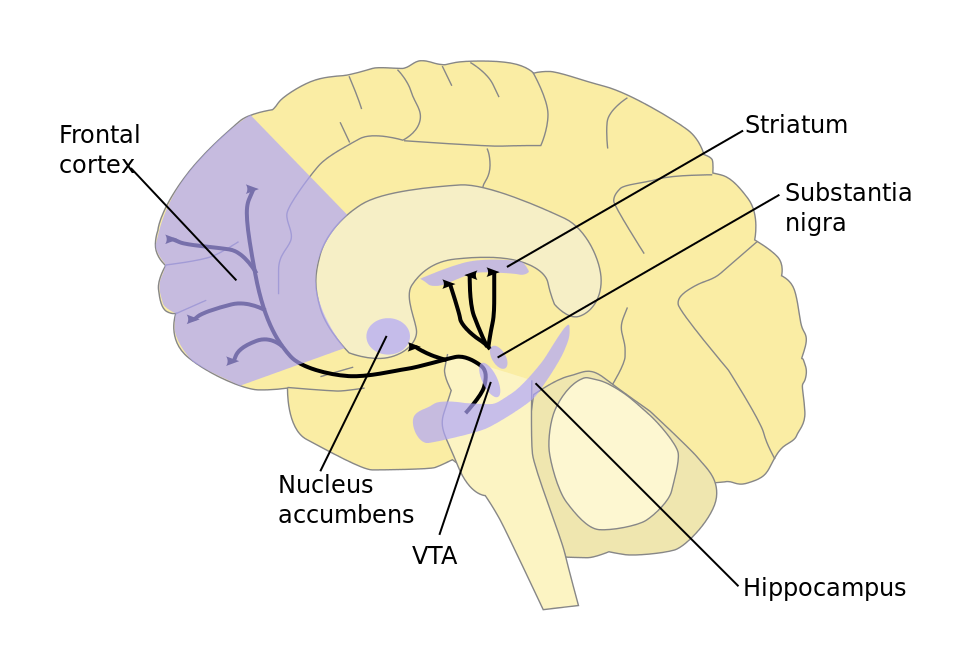
A diagram of a brain in profile showing the location of the substantia nigra
Via Wikimedia
The striatum is known for its role in movement coordination and most of the neurons here (90-95%) are these medium spiny neurons. Research on movement coordination and motor dysfunctions often focus on the medium spiny neurons, but these neurons are regulated by cholinergic interneurons (ChIs; "cholinergic" refers to their responsiveness to acetylcholine and "interneuron" signifies that the neurons stays within a particular brain region). ChIs are massive cells that only make up 1-2% of the neurons in the striatum, but ChIs send out a web of axons to make hundreds of thousands connections per cell. Some previous studies have linked Chl activity with Parkinson’s disease, though the details have been lacking.
In April, Yuan Cai and his colleagues published a study that explored whether ChIs could be manipulated to restore motor functions in Parkinsonian mice. Medium spiny neurons and ChIs talk to each other, so the group wanted to see if ChIs are altered in Parkinsonian mice. The researchers modeled early-stage Parkinsonism by partially damaging a small part of the mouse brain, somewhat resembling how neurons die in people with Parkinson’s disease. Initially, they found no changes in ChIs.
Then, instead of only passively recording from ChIs, the researchers pivoted to recording the ChIs's responses to dopamine and they saw an abnormality. They mimicked natural dopamine input to the area by stimulating dopamine releasing cells. They saw a longer pause in ChIs activity (less electrical changes and therefore less communication with other cells) in the Parkinsonian mice. Cai and his collaborators realized that the abnormal ChI activity was because these cells are unable to detect glutamate — another neurotransmitter released by dopamine secreting cells.
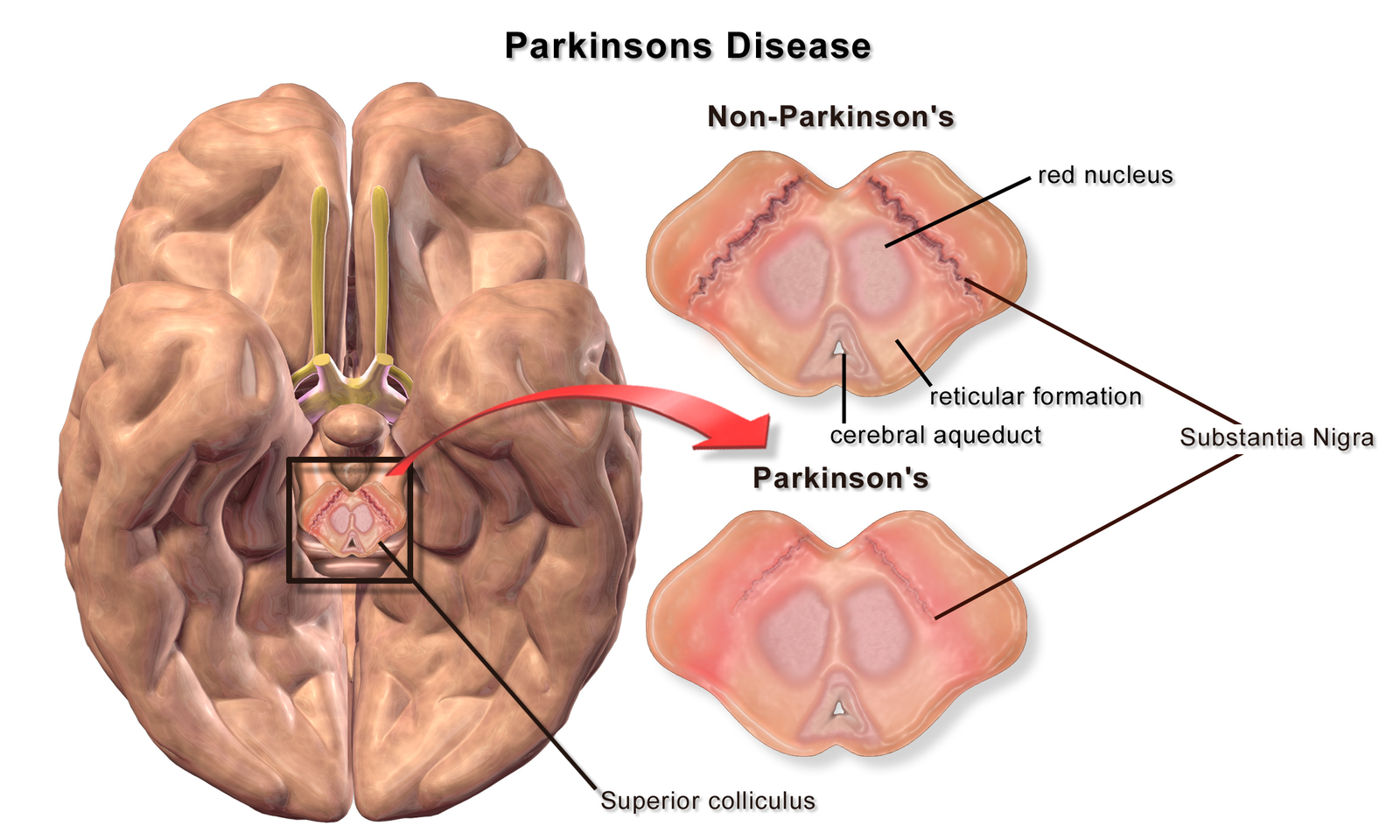
A bottom-up diagram of a brain of a patient with Parkinson's disease illustrating loss of pigmented cells in the substantia nigra
Via Wikimedia
Additional tests confirmed that rather than glutamate availability, the receptors detecting glutamate was altogether missing. For example, Cai and his colleagues measured ChI responses in animals that had reduced glutamate, but did not see the abnormal ChI pause. This meant that glutamate availability was not the culprit. They also found that glutamate receptor mRNA levels (the amount of available blueprint to make a protein) was lower in the ChIs of their experimental group, hinting at less available glutamate receptors. The researchers then detected lower glutamate triggered signaling within ChIs, meaning cells were making less of a fuss when glutamate was detected. When the number of glutamate receptors was increased, ChI activity was corrected back to normal.
When studying the animal as a whole, manipulating ChIs to produce more glutamate receptors dramatically improved the behavioral outcomes Parkinsonian mice. The experimental mice group that usually developed Parkinsonian motor deficits with asymmetrical forelimb movement (measured by comparing when a mouse uses their left or right paw for reaching up, which they normally do equally with either paw) and poorer balance when trotting on a rotating wheel had movements similar to healthy mice when more glutamate receptors were made.
The preventative efforts reported in this paper are extremely exciting, however this is still an understudied cell in relation to motor abnormalities. The preview of this paper lists several important questions that scientists should answer before escalating the importance of glutamate and dopamine release in movement for clinical applications. Are glutamate and dopamine the only molecules released, or can dopamine be released with other molecules to influence movement?, And, is glutamate detection altered as described in human patients with Parkinson’s? Do the results translate from mice to humans?