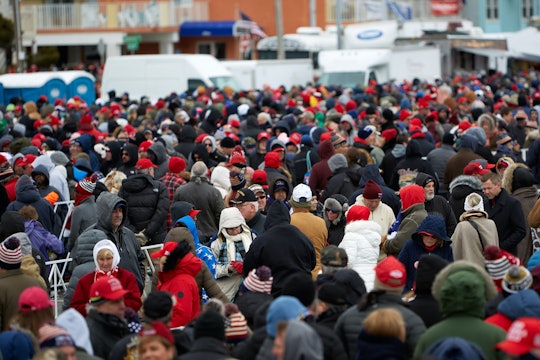
David Todd McCarty on Unsplash
Don't bank on herd immunity to save us from COVID-19
The best way forward is to use quality research to shape policy
There has recently been some speculation that the human population, or at least some segments of it, may already have had sufficient COVID-19 infections to achieve the protective effect of “herd immunity.” There are also new studies using computational modeling that suggest that the population levels of immunity needed for broad protection are lower than the most common estimates of 60-66% immune. While these new and hypothetical constructs of infection-acquired herd immunity show useful directions for the future of public health research for both COVID-19 and other infectious diseases, there are still too many unknowns to use these numbers to design active health policy.
As I wrote earlier this year:
Herd immunity refers to the protection that an at-risk individual can gain by being surrounded by others who are already immune to a disease. It relies on a proportion of individuals within a population already having immunity to an infection, but the exact proportion of individuals needed for herd immunity depends on the characteristics of each particular infection. In addition, the understanding of the concept has been developed mainly as related to vaccination, where immunity is produced in a person by giving a vaccine, rather than that person having to develop and recover from the disease.
Many hard hit communities, such as the Hasidic community in the Borough Park neighborhood of Brooklyn and other urban neighborhoods in London and Mumbai, have already had a substantial number of infections within distinct spatially contained groups, leading people to speculate that they may have established a protective level of immunity within these areas. In addition, many researchers have developed mathematical models of the outbreak and have come up with values lower than the typical estimates of the population needed for herd immunity for COVID-19, ranging from about 43% to as low as 10-20%.
The classical calculation of herd immunity is based on the infectivity of the virus in question, defined by the mathematical expression, 1-(1/R0). R0 (“R-naught”) is the “basic reproductive number” of the virus, which is an indicator of how easily an infection is transmitted. This is an estimate of the number of secondary cases generated by an infectious individual at the start of a novel outbreak, when the rest of the population is susceptible. There are many difficulties in estimating R0 during an active outbreak, resulting in some wide variations in estimates over time and data coming from different geographic locations. Early WHO estimates turned out to be too low, but the most widely used estimates R0 for SARS-CoV-2 now remain at around 2.5 to 3, meaning that one infectious person will infect 2.5 to 3 others. The calculated estimate based on an R0 of 2.5 to 3 results in 60-66% percent of people needing to have immunity before there is any “herd” immunity effect for the population.
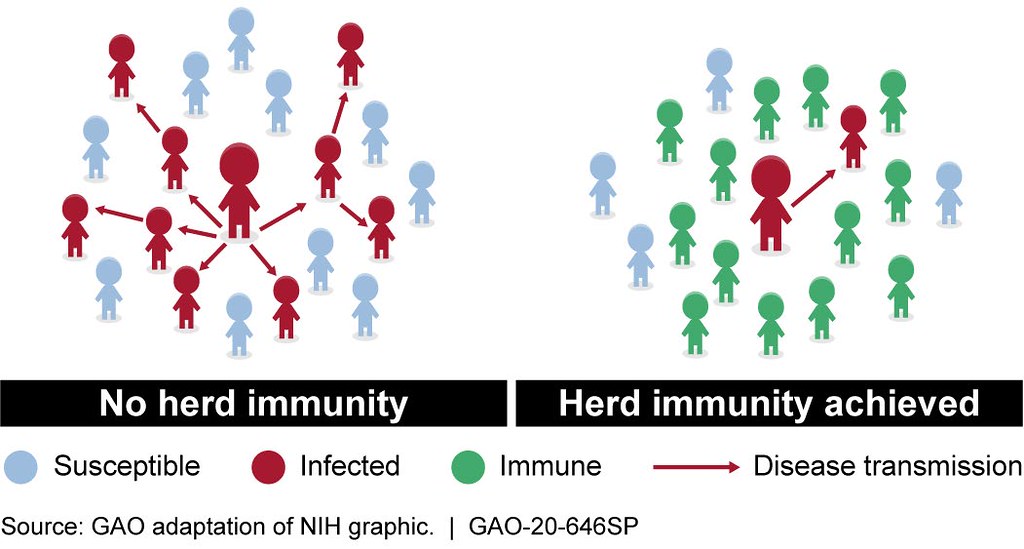
Herd immunity helps reduce the likelihood of disease transmission from infected individuals to non-immune individuals. Immunity can be acquired from vaccines or, in many cases, previous infection and recovery from the infection.
U.S. Government Accountability Office on Flickr.
A mathematical model recently published by Tom Britton and colleagues in Science suggests that because population groups vary by factors including age and rates of social activity and contact, herd immunity could be established through illness and recovery with only around 43% of the population, instead of the 60% required using a classical model assuming an R0 of 2.5.
While this type of mathematical model for herd immunity is theoretically interesting since it attempts to capture contextual factors and elements of population heterogeneity, it is still too early to be directly applied to public policy. Our current knowledge about SARS-CoV-2 (the virus that causes COVID-19) is incomplete, including a lack of information about how we may develop immunity and how long immunity will typically last. Mathematical modeling is also based on broad assumptions that are often untested in the real world. Much more research is needed before we know if these new ideas about herd immunity should be applied to public health interventions and planning.
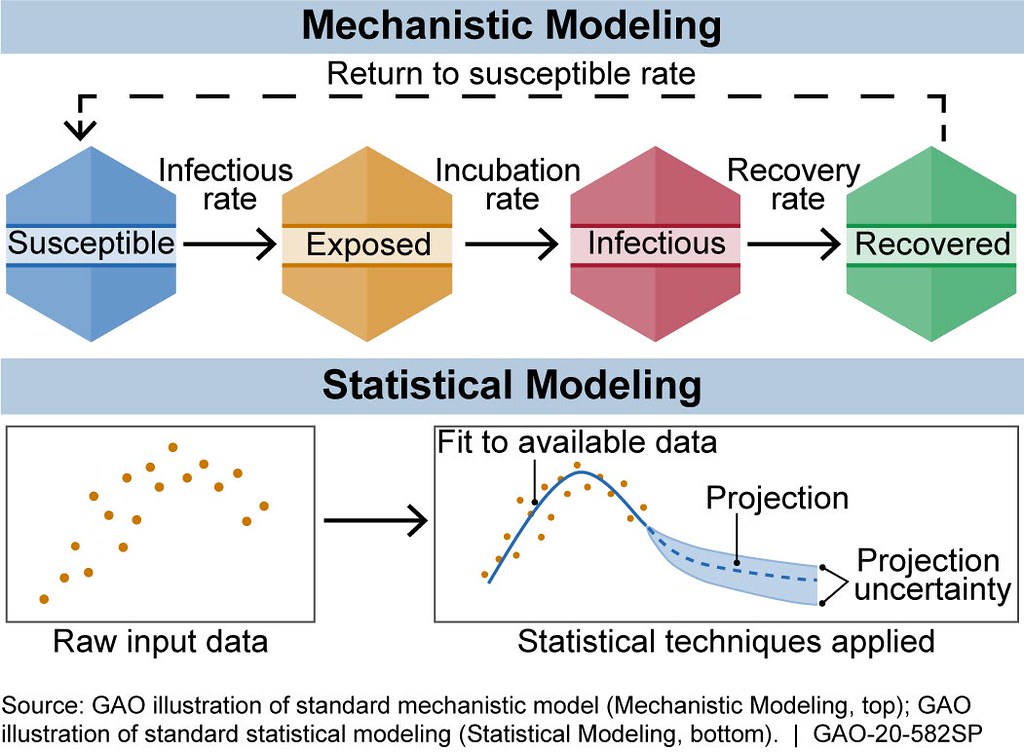
There are generally two broad categories of infectious disease models: mechanistic models, which use scientific understanding of disease dynamics and human behavior, and statistical models, which rely only on patterns in the data
U.S. Government Accountability Office on Flickr.
The most striking example of how fast our understanding can change is the recent confirmation of reinfection with a second case of COVID-19 after four and a half months in Hong Kong. Unlike earlier reports of reinfection, which were mainly anecdotal, this case was confirmed based on viral genome sequencing, showing that the second infection was from a genetically distinct strain. This suggests that reinfection is an important possibility and that immunity acquired through illness and recovery may last only months. This new case adds additional elements of uncertainty to Britton and colleagues’ model, since the authors state that their current model was designed based on the assumption that “infection with and subsequent clearance of the virus leads to immunity against further infection for an extended period of time.”
Reinfection and the typical duration of immunity are not the only uncertainties. It also remains unclear to what degree immunity is antibody-mediated versus cell-mediated, which kinds of antibodies are most important, whether immunity might prevent future disease or only make reinfections less severe, and whether prior exposure to common cold coronaviruses offer any protection. The immune response also may depend on characteristics beyond age, including biological sex and individual genetic variation, and other factors. The data available is often incomplete, meaning that mathematical models may be based on biased samples; underreporting of data has been high, and areas without sufficient testing do not provide adequate data.
There are also a number of difficulties inherent in using the basic reproductive rate to predict disease spread, and it is difficult to disentangle the basic rate R0 from the actual transmission rate (Rt), which is impacted by changes in behavior and in population immunity over time. If done properly, all of the measures meant to control the virus, including lockdowns, social distancing, business closures, travel bans, mask-wearing, and contact tracing, will reduce the transmission. While this is a good thing for the public’s health, it makes the data collected ambiguous: has disease transmission has been slowed by public health measures, or is it waning naturally?
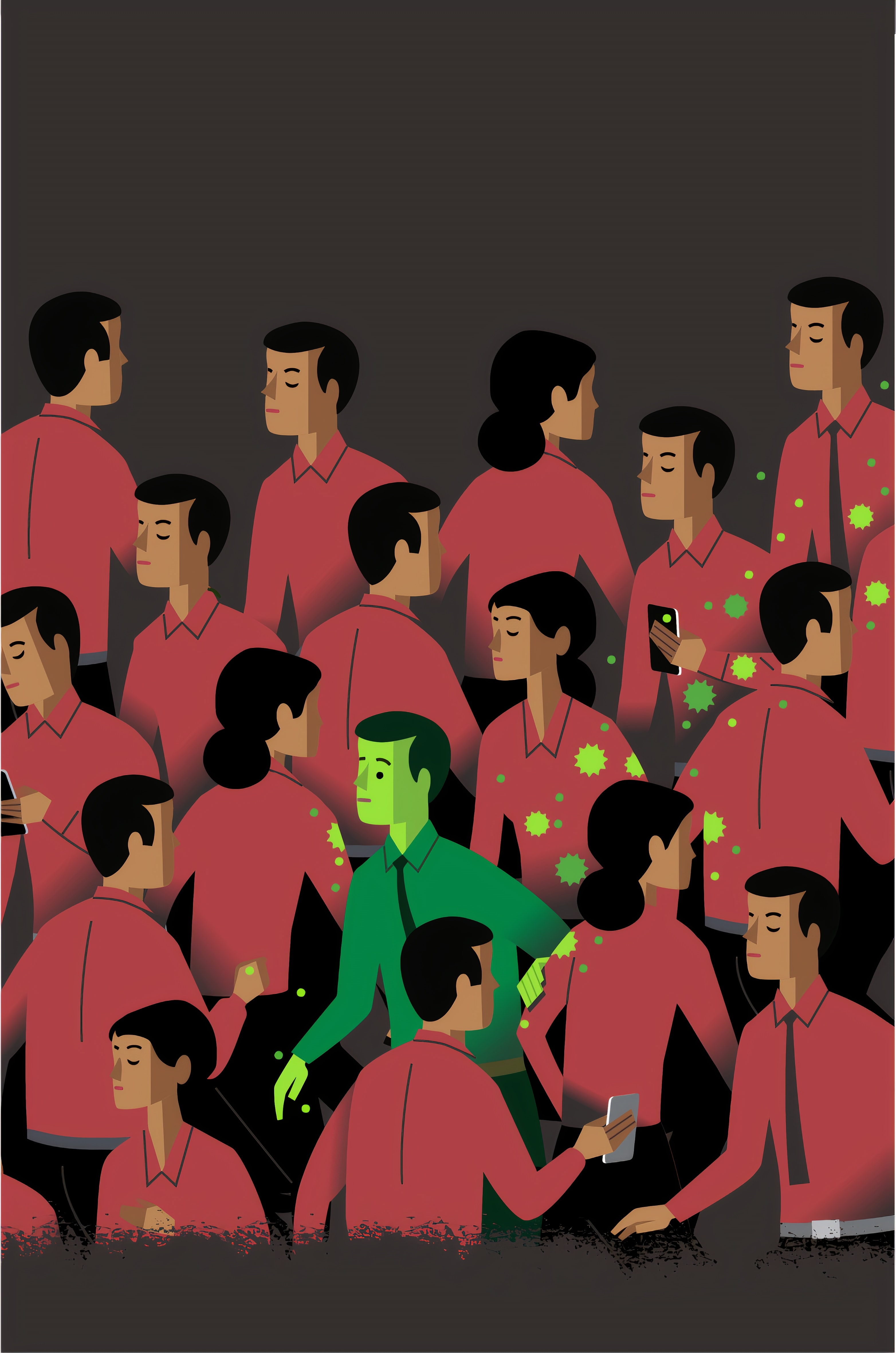
Current estimates suggest that a person infected with COVID-19 will, on average, pass the virus to 2-3 other people.
United Nations COVID-19 Response on Unsplash.
There are also speculations that the amount of virus a person contacts impacts the severity of illness (this is known as a dose-response relationship), potentially explaining why masking is effective. It is still unclear to what degree seasonality plays a role in transmission, and more research is needed on the exact mechanisms of virus spread and persistence in the air and the role of indoor conditions such as humidity, temperature, and ventilation. Finally, once a vaccine becomes available, it will impact herd immunity, though the results will depend both on the effectiveness and the distribution of any future vaccines as well as whether people are willing to get the vaccine at all.
Throughout this pandemic, the concept of herd immunity has been frequent fodder for wishful thinking. Some countries, including Britain and Sweden, attempted to rely on herd immunity rather than implementing broad control measures. Now Britain has reconsidered this plan, and Sweden has sustained much larger spread of the disease and greater number of deaths than its neighbors.
In the United States, wishful thinking about the virus disappearing on its own has delayed needed intervention and prompted premature reopening. Pandemic control measures have many unpleasant side effects, and herd immunity can be an appealing concept for those who seek reassurance that the world will eventually return to normal, but our best way forward requires an understanding that conducting quality research and applying it effectively to policy take time and a great deal of work.