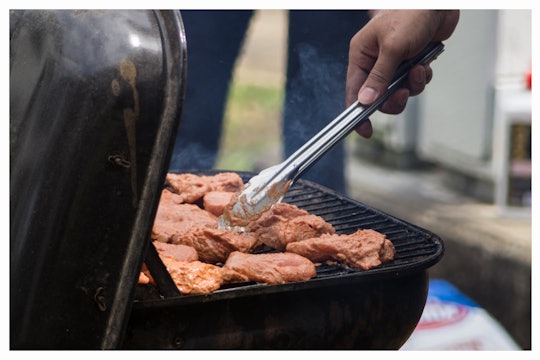
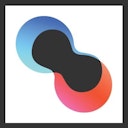
Produced in partnership with New Harvest
From muscle to meal, this is the journey meat takes
There's complex chemistry happening every time you throw burgers on the grill
Pork, beef, lamb—we tend to closely associate raw meat with the animal it comes from. Yet from the moment of slaughter, irreversible chemical changes take place in the dead animal to create the meals we know.
In fact, the journey from muscle to meat begins even before the slaughtering process. For instance, so-called "pre-slaughter stress" can dramatically affect meat quality. In pigs, this process is referred to as pale soft exudative meat, resulting in meat that is a very pale color and that tastes particularly acidic. In cattle and sheep, the equivalent is the self-explanatory Dark Firm and Dry Meat. Both defects cause the meat to be less valuable and unpopular with consumers. They happens because stress—perhaps caused by handling or stunning—affects the chemistry of the meat after death.
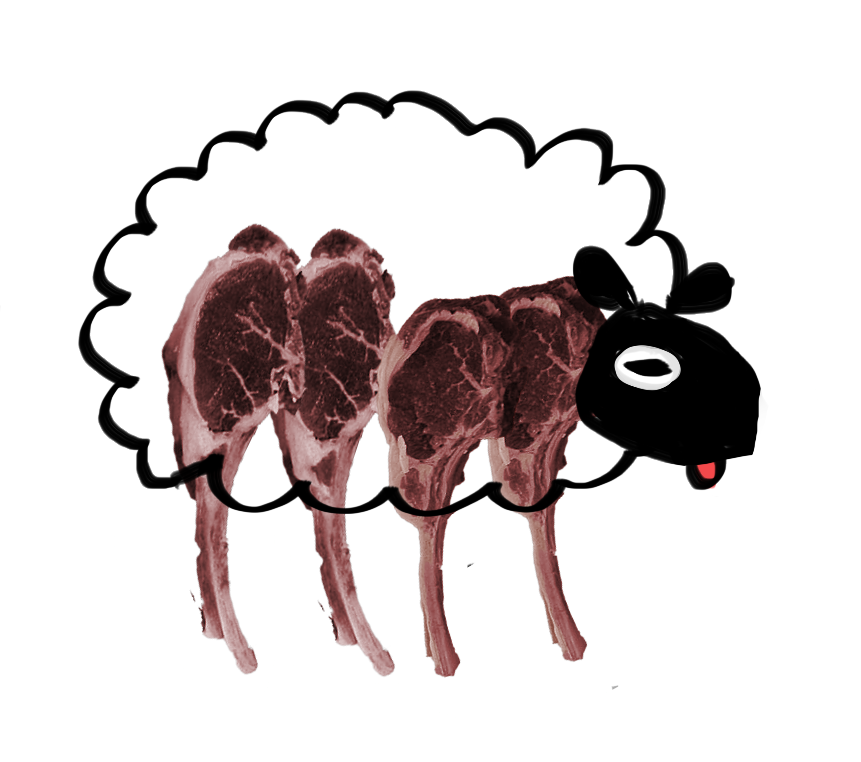
Typically, the reddish color of meat is determined by myoglobin, an oxygen-binding protein in muscle cells, similar to the hemoglobin in your blood. The concentration of myoglobin determines the color of the meat—that's why myoglobin-rich beef is deep red and myglobin-poor poultry characteristically white. When unstressed animals die, lactic acid builds up in the muscle cells, slowing their mitochondria activity. This leaves surplus oxygen (which would normally be consumed by the mitochondria) for myoglobin to bind with. The oxygenated myoglobin then turns a bright red color (think hamburgers). But when stressed animals burn through their glycogen stores, they have none left after death to produce lactic acid. Their mitochondria keep going like gangbusters, using up the muscles' remaining oxygen, and leaving myoglobin unbound—causing the meat to turn an unsightly purplish-pink color. This kind of discoloration costs the U.S. meat industry $1 billion dollars of revenue every year.
Meat undergoes further chemical and structural changes during cooking. As you may have noticed, meat changes both color and texture when warmed, going from red to gray to brown. These color changes are influenced by a few things. What myoglobin—the oxygen-binding protein in your muscles—is doing plays a big role. Myoglobin carries an iron atom which in turn carries oxygen, like your hand carrying a cup which in turn contains tea. Myoglobin that carries a lot of oxygen is a bright pink (like the color of ground beef, which gets mixed with air during grinding). When meat is exposed to air, the iron in myoglobin essentially rusts, spilling its oxygen, causing the meat to turn brown. Heat also denatures meat proteins. When cooked, myoglobin separates from its iron and forms a "hemichrome" pigment, also brown/tan-ish. When myosin and actin, muscle-movement proteins, are cooked, they unfold and form intricate knots—a similar chemical process occurs when you cook an egg. And, like an egg, meat becomes more firm during this denaturation process. After prolonged heat, collagen, the main protein in connective tissue, also breaks down to gelatin, converting cartilage into melting jelly.
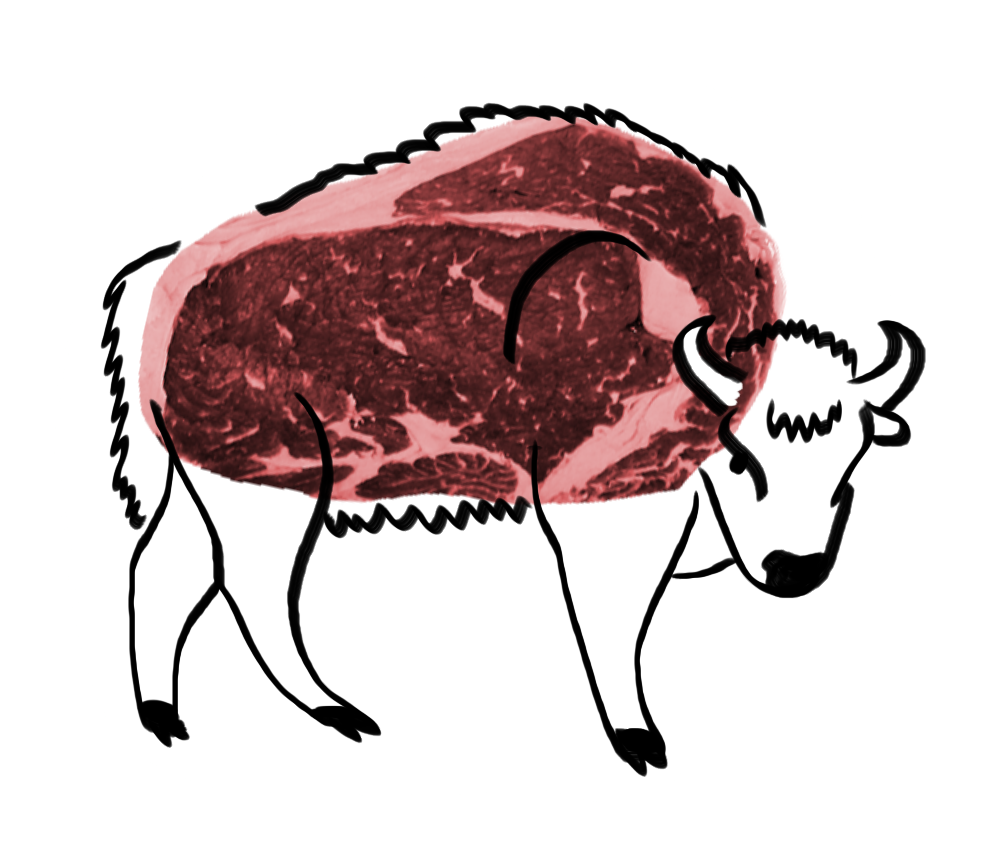
Meat's shape is also altered by the loss of water during cooking, which typically causes it to shrink in size. This in itself leads to a drier product—but since fat also melts under heat, the perception of juiciness depends on the cut's proportions of fat and water. In fact, emulsions of fat and water are stabilized by proteins, such as myosin, which are more stable under high temperatures. This higher stability allows more water to remain in fatty meat than in lean meat while cooking. So, a cooked fatty cut of meat will have more water than a lean cut, once both are on the dinner plate.
Beyond these textural changes, meat goes through a series of taste-modifying chemical reactions as temperatures increase. During the Maillard reaction, usually occurring between 140 and 165°C, amino acids (for instance from degraded proteins) react with sugars to form thousands of different compounds, each with its own unique flavor. At higher temperatures, caramelization breaks down the structure of sugars and produces an even greater abundance of flavorful molecules. Finally at extremely high temperatures, pyrolysis, or charring, produces carbon from the complete breakdown of surface molecules. All of these changes contribute to an arguably more complex flavor in cooked food than you can often find in raw food.