Deborah Jin engineered new quantum states of matter — twice
According to colleague Kathryn J. Levin, Jin "probably would have gotten the Nobel"
“She probably would have gotten the Nobel.” University of Chicago physicist Kathryn J. Levin doesn’t hesitate when asked about fellow physicist Deborah S. Jin, affectionately known as Debbie.
She doesn’t have to try hard to make her case: during her short career, Jin was honored by one award after another. She invented new experimental techniques that led her to engineer new quantum states of matter twice, and her experiments were inspirational to many other physicists. Some of her work directly influenced theorists working on complex problems such as that of perfect electrical conductivity. She was a quiet yet strong and unforgettable presence wherever she went and a dedicated mentor that sought to lead by example.
Jin was born in California in 1968 and grew up in Florida where her father worked as a physics professor. Her mother and brother also studied physics. Her husband, physicist John Bohn, later wrote that she grew up “in a home with physics in the air.” She fully leaned into it and her undergraduate work at Princeton University earned a prize for experimental physics.
.jpg)
Deborah Jin in one of the lobbies of the JILA facility on the CU-Boulder campus
Glenn Asakawa (University of Colorado) via Wikimedia Commons.
She received her PhD from the University of Chicago where she studied materials that perfectly conduct electricity (called superconductors). There, Jin learned about particles called fermions that later defined a large part of her career. In 1996, she moved to JILA, a research institute at the University of Colorado Boulder. In Colorado, Debbie shifted her focus to the sub-field of atomic, molecular and optical (AMO) physics.
AMO physicists study atoms, molecules and light and they devise ways to control them. Often, they work with ultracold systems — close to absolute zero (-273° C) — which obey quantum mechanics. The toolbox of AMO physicists mostly contains lasers and magnets. Interactions between laser light and atoms result in electric forces whereas interactions between atoms and magnets give rise to magnetic forces. Physicists use these forces to make atoms ultracold, as close to absolute zero as a trillionth of a degree, and then keep them stuck in place so their properties can be measured.
The JILA research team that Jin joined, led by Eric Cornell, had collaborated on engineering the first ever Bose-Einstein condensate (BEC), work that earned a Nobel prize in 2001. A BEC is a quantum state of matter that forms when particles classified as bosons are made extremely cold. At extremely low temperatures, they all assume the same, lowest energy state and behave as a single chunk of quantum matter rather than thousands of separate particles.
In the late 1990s, ultracold bosons and BECs were the big sensation at JILA. As a postdoctoral researcher, Jin worked with ultracold bosons for the first time in her career and had to learn a whole new set of experimental techniques to do so. She undertook “total retraining,” according to Levin. Jin learned quickly and soon made important contributions to AMO studies.
.jpg)
A vacuum chamber apparatus that captures cloud of ultracold atoms in a Bose-Einstein Condensate (BEC), which form a few micrometers below the glass pieces within the chamber.
E. Cornell group (JILA) via Wikimedia Commons.
In 1997, she was permanently hired at JILA and started her own research group. “She was starting in a field where the doors had been widely open,” Levin notes, and she decided to open them even further. Jin set out to make fermions ultracold.
Every particle in the universe is either a boson or a fermion, depending on its spin. Two fermions cannot occupy the same quantum state so they cannot all “fall” into their lowest energy state at once. In other words, coaxing them into being very cold is very difficult. At the time, this was considered a huge experimental challenge. Creating an ultracold fermion gas would constitute an experimental breakthrough and Jin would be engineering a brand-new quantum state of matter. A year and a half later, Jin and her first graduate student succeeded in doing exactly that.
Despite becoming an AMO experimentalist only a few years earlier, the techniques Jin developed on this project were more successful than those competing research groups were using. She started from scratch, inheriting an empty room instead of a full lab. “I started making circuits on the floor of my office” her first graduate student, Brian DeMarco, recounts about their early days.
They also built their science from scratch, innovating everything from how to produce atoms they wanted to cool to new measurement techniques. Jin was not intimidated by this challenge. Her determination paid off: the 1999 Science paper where she and DeMarco reported the success of this experiment has thousands of citations and contemporary AMO physicists now routinely engineer and study ultracold fermion gases in their labs.
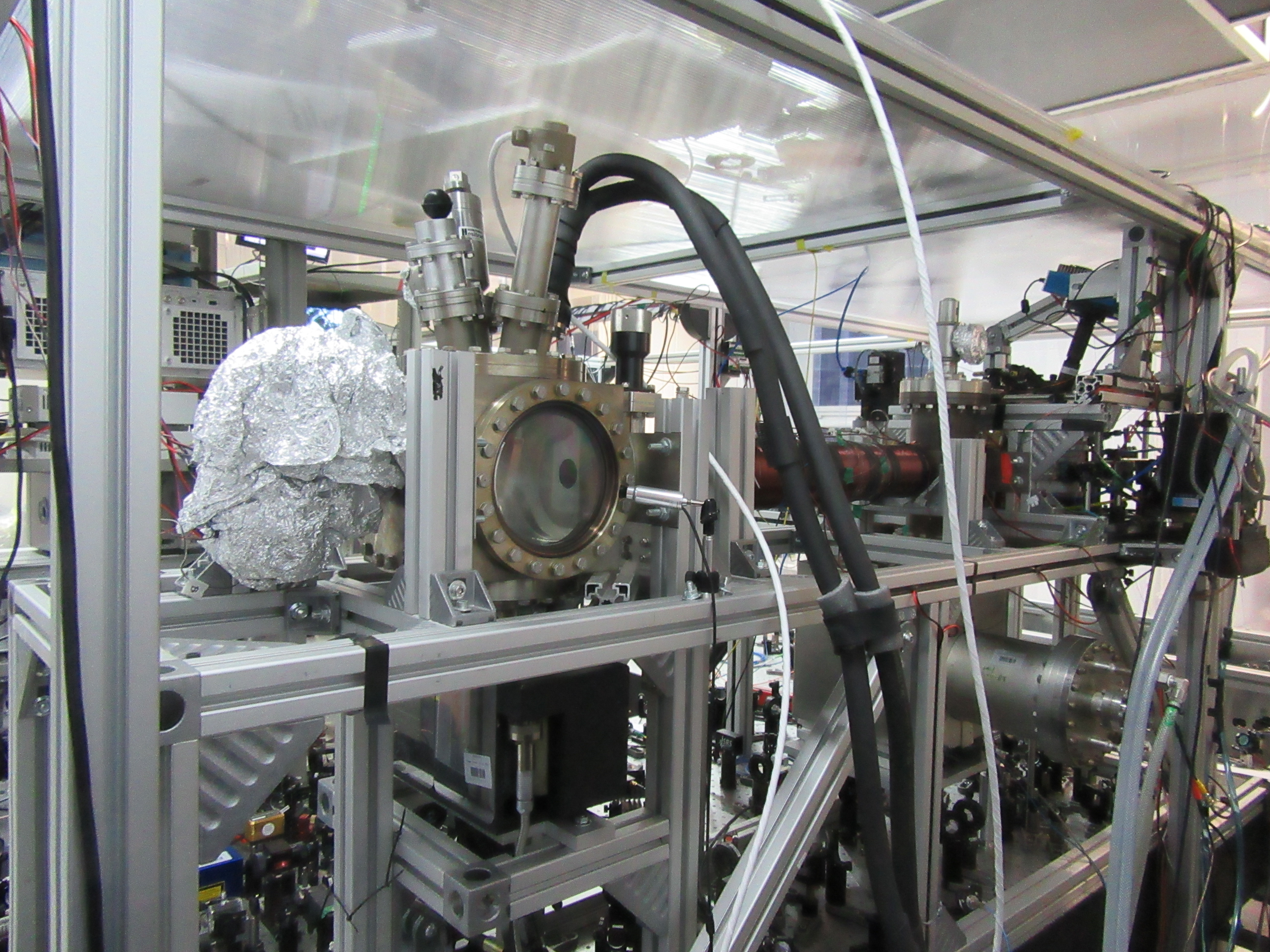
Equipment for obtaining and researching ultracold atoms, located at the National Laboratory of Atomic, Molecular and Optical Physics in Nicolaus Copernicus University in Toruń, Poland.
Ciacho5 on Wikimedia Commons
In 2001, Jin and her research team experimentally pushed fermions to form pairs that then behaved like bosons. Their experimental achievement advanced theories about fermions and superconductivity. One famous mechanism of superconductivity, recognized by the 1972 Nobel prize, requires that electrons, which are a type of fermion, pair up. However, details of this pairing process are not understood for all superconductors. Jin’s experiment offered theorists a new setting for figuring out how fermions can act as bosons and how that can result in special properties like superconductivity.
Levin, who has spent years studying odd superconductors, recalls Jin’s work inspiring her to start collaborating with AMO researchers herself. Other physicists started to turn to ultracold atomic systems to emulate models they could previously, due to their complexity, only study by solving equations or using supercomputers.
In 2008, Jin and JILA colleague Jun Ye helped launch the study of ultracold quantum chemistry by making diatomic polar molecules ultracold instead of atoms, effectively engineering another new kind of quantum matter. Once they are ultracold, chemical reactions between molecules follow the rules of quantum mechanics, but cooling molecules to extremely low temperatures is more complicated than cooling atoms. There are many more ways for molecules to get energetically excited and consequently warm up.
Jin died of cancer in 2016. She was only 47 but her legacy is tremendous. Today, engineering an ultracold fermion gas is a skill AMO physics graduate students often learn early on in their doctoral training. Years after his first taste of ultracold fermions, DeMarco is mentoring his own students in studying them in new, complex configurations. Levin also continues to collaborate with AMO experimentalists; such collaborations have become more common since the early 2000s. And many physicists continue to work with ultracold molecules. Many ripples are still spreading from the successes of Jin.
It is not a coincidence that Jin’s success has been part of the incentive for physicists to collaborate more with their theoretical or experimental colleagues as well as across different branches of physics. Openness to collaborative work was a part of the research culture at JILA and Jin championed this approach to doing science. As DeMarco recounts, she had also been known to be a kind and generous team leader.
Working in a male-dominated field (women comprise less than 20% of physics faculty in the United States), Jin cared a lot about the status of women in physics. She was committed to leading by example and being a mentor. This way of addressing the gender disparity in physics was best suited to her strengths. “When invited to events aimed at improving the participation of women, she’d always do it,” DeMarco recalls, “and she’d meet with young women scientists all the time.” She had particularly appreciated the L’Oreal-UNESCO for Women in Science Award she received in 2013.
Her determination and careful approach to doing science made Jin a great role model for all young scientists. She believed that “unless physics and nature say no,” clever and hard work could get her through all setbacks, DeMarco recalls. But this conviction did not make her quick to jump to conclusions. “I learned from her to be skeptical of your own work,” DeMarco underscores. Jin really wanted to explain her work clearly and did not claim any result she was not absolutely certain of.
Following her passing, Bohn wrote that Jin encouraged young physicists to be like a fermions and pursue their own interests and individuality. She herself was quite like a fermion too: she could not be forced to assume the same state as everyone else. She was always driven to do things her own way and did not fear setting precedent. A true science hero, she used that drive to advance the field she worked in and move its community forward.