Scientists are mapping the genetic tool fancied a 'fountain of youth'
Telomerase protects our genetic information as cells copy DNA. It's stymied scientists for decades
If you hear that a story is about “protecting your genetic information,” you might expect another cautionary tale about protecting your genetic code from insurance companies, employers, or the government. But each cell in your body also needs to protect your DNA from another threat – yourself.
Your DNA is stored in long, coiled strands called chromosomes, warehoused in a compartment called the nucleus. Just as libraries have archivists to protect valuable books, your cells have “archivists” in the form of telomerase, a molecular complex of proteins and RNA that protects the ends of your chromosomes. After decades of trying to catch a glimpse of it, scientists now have a better idea of what this guardian of your DNA looks like and how it works.
As any good librarian will tell you, information should be shared, which is why scanners and copy machines are mainstays of libraries. Cells have their own Xerox machines – molecules called polymerases, which copy DNA in a process called replication. When a cell splits in two, polymerases latch onto DNA and use one strand as a template to stitch together the complementary bases of a second, ensuring each cell gets a full set of genetic information. They're great at their job, but during the copying process they use RNA "primers" that are erased and rewritten in DNA – all except the last, which can't be replaced. As a result, each time DNA replicates, the ends of chromosomes get shorter – as though the Xerox were running low on ink and cut off the end of a page with each copy. Any unique genetic information on this cut-off portion is lost. To prevent this, a special polymerase, called telomerase, adds “generic” caps of DNA called telomeres to the ends – like adding gibberish to the ends of books. If the caps are shortened, no unique information is lost, just gibberish.
The 'fountain of youth'
But the gibberish isn't random - instead of blindly pressing keys on a keyboard, telomerase "copies and pastes" a short DNA sequence over and over, based on an RNA template it contains. This sequence serves as a launch pad for telomerase to latch on, make a copy, hop off, then do it all over again, lengthening the chromosomes. The telomere sequence also provides docking for “watchguard” proteins, which help cells distinguish between the ends of chromosomes and breakages, so that cells don’t try to “fix” breaks by fusing regions of DNA that should be separate (such fusions can lead to cancer).
Telomerase is typically only expressed in cells that need to replicate a lot, like stem cells. For cells that don’t have to replicate as much, the caps gained at “birth” are long enough to protect them for life. But eventually they get too short, which can cause cells to die or go into a state of hibernation, called senescence, to protect your genes – this is thought to be a cause of aging. Some people have therefore looked to telomerase as a molecular “fountain of youth,” reasoning that if we could get it working in adult cells, we could potentially elongate life.
However, there’s good reason why telomerase isn’t expressed in all cells – allowing cells to replicate without end isn’t good. In fact, it’s one of the defining characteristics of cancer (up to 90 percent of cancer cells express telomerase), and anti-telomerase drugs have been proposed as cancer treatments.
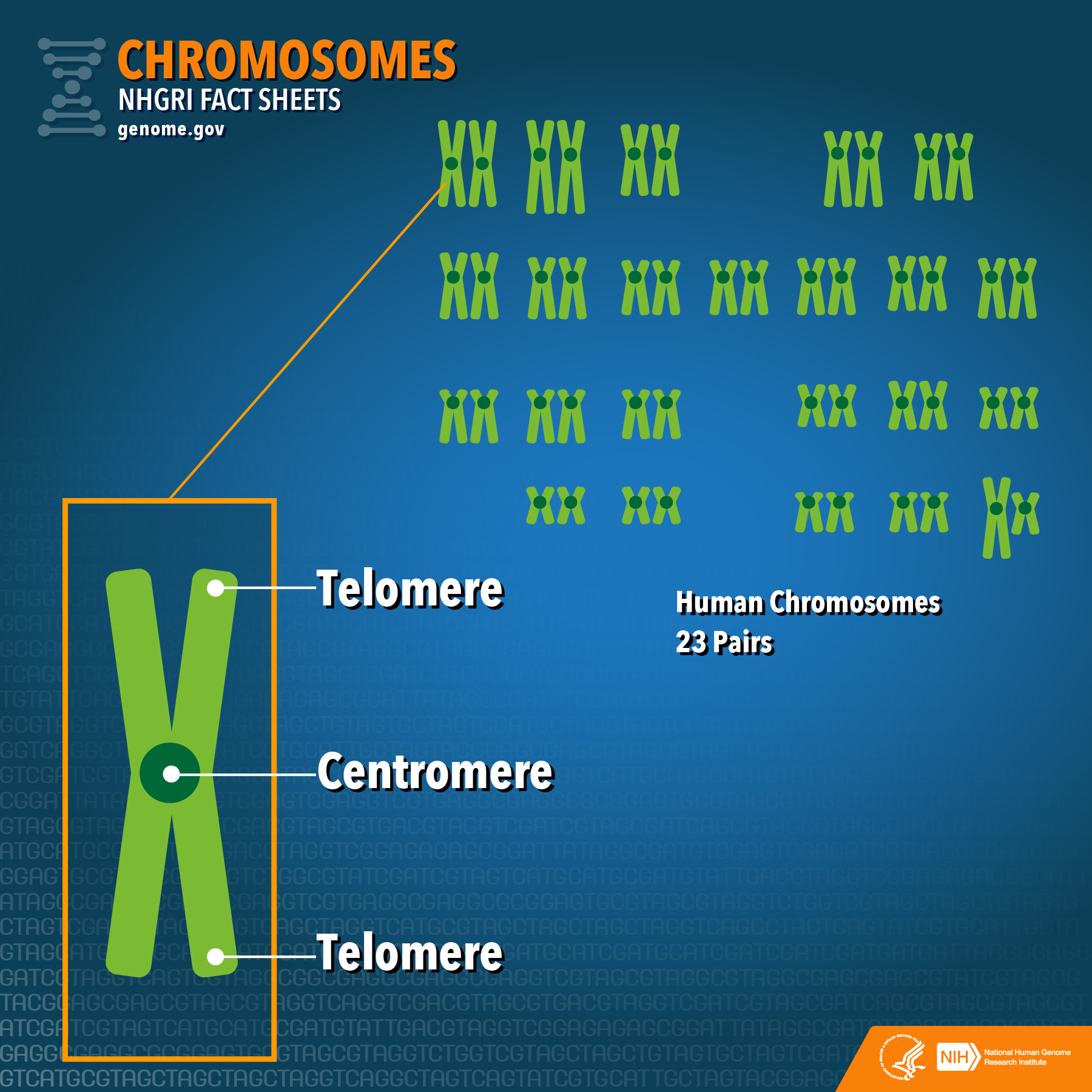
Scientists have been working for decades to try to deduce the structure of telomerase, but it's proven very hard to study. We didn't know how these subunits fit together, how many there were, or even what made up the subunits. The challenge faced by a group of researchers at UC Berkeley, led by Kathleen Collins and Eva Nogales, was like they had ordered a build-it-yourself kit from IKEA, which arrived without instructions or pictures – just a bag of parts, which might have the wrong number of parts or parts from other kits.
To figure out which parts they needed, a postdoctoral fellow, Thi Hoang Duong Nguyen, over-expressed two components they knew were involved – the polymerase protein TERT and the RNA template hTR – and gave a molecular tag so that she could pull them out of cells with the molecules they were bound to, which she then identified. At this point, the team didn’t have the instructions, but they at least identified the parts.
Seeing what's never been seen
In need of a guide, the team tried to take a "picture" of their own. Telomerase is very flexible and dynamic, great for navigating cells and adding caps to chromosomes, but bad for studying with a classic tool of structural biology: X-ray crystallography. In this technique, scientists shoot a beam of X-rays at a molecular crystal. When the rays hit the molecules, they bounce off at different angles and hit a screen, producing a series of spots called a diffraction pattern, which scientists use to trace the rays back to their starting points.
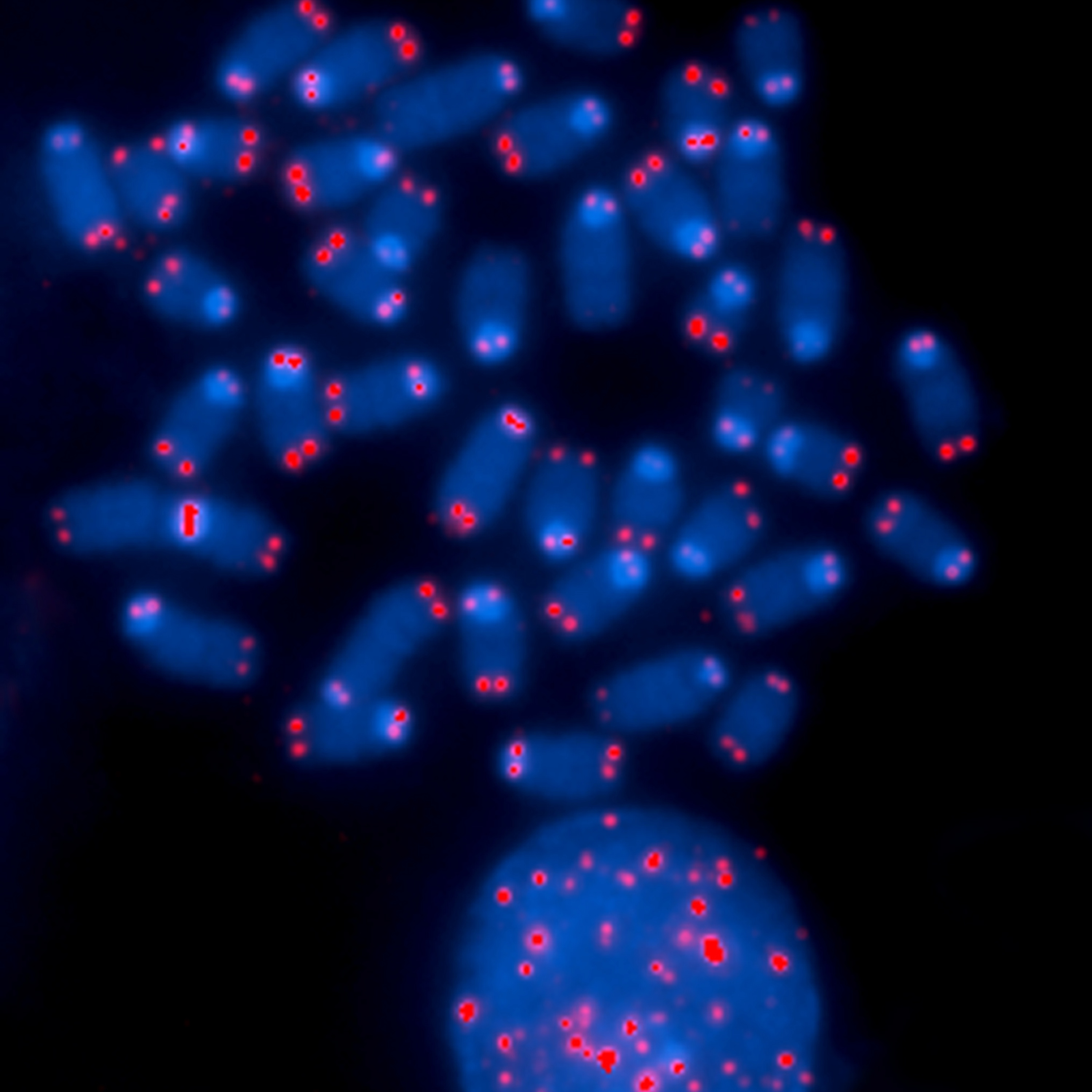
NIH Image Gallery / Flickr
Imagine you have a large crowd of identical people, all doing jumping jacks, and you want to see what the people look like. In crystallography, you need to get all the people jumping in sync and to freeze them long enough for a picture. Because the jumpers are identical and in identical positions, you can get a high-resolution “picture” – you can clearly distinguish the positions of different atoms – but only of this pose. Without more information to help you interpret the picture, it can be hard to know what's going on.
It’s difficult enough to get a single molecule to freeze, let alone a group of connected molecules, so crystallography is usually limited to single proteins, parts of proteins, or tightly bound molecules. Telomerase does not fit the bill. Using crystallography, scientists had been able to get a sense of some subunits, but not how they interacted.
Enter single-particle cryo-electron microscopy (cryo-EM), which is better suited for wiggly molecules like telomerase. While electron microscopy isn't new, recent advances in cryo-EM technology have drastically improved its ability to see structures. In this technique, instead of making the molecules "freeze" themselves, the researchers freeze them in a thin film of clear ice. Electron microscopes work much like light microscopes but, instead of using glass lenses to focus light, electron microscopes use magnetic fields to focus beams of electrons to get an image. Electrons have a much shorter wavelength than visible light, so provide much finer detail – if you don't fry your sample in the process (a short wavelength means high energy). This is where the cold comes to the rescue, preventing radiation damage.
This gives researchers a bird's eye view of the "crowd," from which the researchers can identify particles and average their pictures. Using lots of computing power, they reconstructed these 2D images into a 3D map of telomerase.
A puzzle of a map
But the scientists, never having had a good look at the complex before, still didn't know how their map corresponded; they had put together the edges of the puzzle but needed help with the center. Through tests, they prevented some subunits from binding and then looked for gaps in the resultant images, revealing where the pieces fit. And once they knew what went where, they could place the higher-resolution images of subunits, gained through X-ray crystallography, into their cryo-EM map.
This showed them that human telomerase is made up of two lobes, glued together by the RNA component, hTR; and they answered the question of how many copies there were of each subunit. One lobe consisted of TERT encircling the template-containing part of hTR and the other lobe contained helper proteins (two sets of a four-protein group and one additional protein) attached to hairpin folds in hTR's other end.
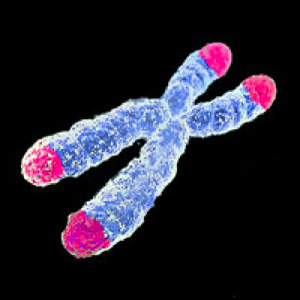
Telomeres on a chromosome
AJC1 / Flickr
Earlier in her career, Collins discovered that mutations in one of these helper proteins, dyskerin, cause a disease called dyskeratosis congenita, and now she can actually see why: the telomerase structure shows that dyskerin is crucial for latching onto other subunits, so single-point mutations can disrupt the entire telomerase complex. Without functional telomerase, the patients have short telomeres and, consequently, problems with tissues that have to regenerate a lot (skin, guts, and bone marrow).
The current structure, although a significant improvement from the previous "glimpse," is still fairly low-resolution. The scientists can see how the subunits fit together, and they can see the basic shapes within the subunits (such as helices and coils) but they can’t see the intricate details of each subunit (like individual amino acids). They’ll need a higher-resolution image to find potential drug-binding pockets that pharmacologists can use to design treatments.
The researchers acknowledge that this paper, though important, is only a midway point: they now have a blurry JPEG of the telomerase, but they’re still looking for a high-res PDF. Hopefully, further updates in cryo-EM technology, complemented by cell and molecular biology experiments, will help them fill out the instruction manual for this potential key to cancer and aging.