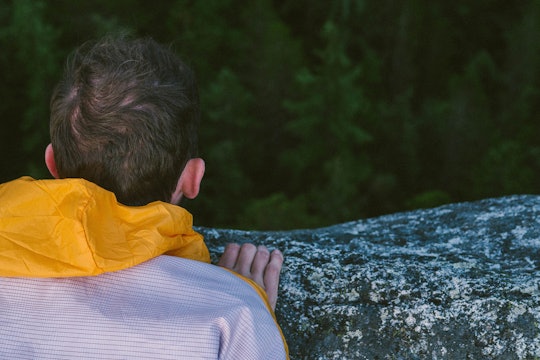
Photo by Greg Rakozy on Unsplash
Haven't heard of RNA therapy yet? You will
After a decade of painstaking progress, the underdog is on the brink of treating a broad range of diseases
Although you may have heard of gene therapy, you probably haven't heard of RNA therapy. Unlike gene therapy, which provides new DNA to cells, RNA therapy modifies or provides ribonucleic acid (RNA) to patients' cells. Despite the overwhelming popularity of gene-editing technologies like CRISPR, new advancements in RNA therapy are poised to address some of their serious limitations. RNA therapy now has the potential to treat a wide variety of diseases, including cardiovascular disease, hemophilia, and cancer.
RNA therapy and gene therapy are often lumped together, but they're actually distinct classes of treatment known as nucleic acid therapies. They're related through the central dogma of molecular biology, an elegantly simple foundation for all living organisms. Outlined by Francis Crick in 1953, the theory states that genetic information flows from DNA to RNA to proteins, in that order. Generally, to transfer information, DNA’s string of chemical units, known as nucleobases (adenine, thymine, cytosine, and guanine), are transcribed to RNA’s nucleobases (adenine, uracil, cytosine, and guanine). A type of RNA called messenger RNA (mRNA) is then translated into protein by a biological cipher, which converts three nucleic acids to one amino acid.
But in the last six decades, we've learned that this transfer of information is actually much, much more complicated. While Crick's diagram suggested RNA's role was limited to carrying genetic information, we now know RNA is an animated, powerful commander of our cells’ machinery. Researchers have identified types of RNA responsible for several important activities, including coding and organizing protein creation, modifying how instructions for DNA are transmitted, destroying other RNAs, and preventing rearrangement of genomes.
By focusing directly on what many refer to as the software of the cell, RNA therapies have direct biological targets: a specifically engineered strand of RNA is delivered to interact or produce specific functions within the cell. And simply tweaking the nucleobase code can adjust what the therapy impacts.
“You simply change the sequence and you’re hitting another indication,” says Professor Paula Hammond, head of MIT’s Department of Chemical Engineering and a member of the Scientific Advisory Board of Moderna Therapeutics. “If the platform works once, it multiplies.”
This makes RNA therapy more targeted and more versatile than conventional treatments, like immunotherapy, or even aspirin or insulin. (In comparison, we still don't know how many drugs — including Tylenol — actually work.)
There are two main types of RNA therapy, antisense – which degrades dysfunctional or harmful proteins in the cell – and mRNA, which produces functional proteins.
Currently, antisense therapy is more common, though more complicated. Numerous mechanisms and classes of RNA are considered antisense, but all focus on the complementary pairing of RNA strands. (For example, a strand of AUCG binds with a strand UAGC; A binds with U, and C binds with G.)
Several RNA antisense therapies already exist to improve kidney transplant outcomes, treat hemophilia, and lower LDL-cholesterol. One such mechanism called RNAi, was a 2006 Nobel Prize-winning discovery, and offers the basis for a plethora of exciting new therapies. RNAi employs special types of short RNA that act as specific instructions to the cell. A protein complex uses these instructions to destroy mRNA that are creating dysfunctional proteins. Alnylam Pharmaceuticals is preparing to launch the first siRNA drug this year, targeting an inherited disease called ATTR amyloidosis, and numerous other companies are racing todevelop RNAi therapies.
The second kind of RNA therapy focuses on replacing mRNA. Cystic fibrosis patients, for example, fail to make a functional protein called CFTR in their cell membranes. Scientists hope to have patients inhale particles containing healthy mRNA, replacing the dysfunctional CFTR protein in the lung. Translate Bio will start selling an mRNA candidate to treat patients with cystic fibrosis within the next several months, and another company, Moderna Therapeutics, is also developing a treatment for cystic fibrosis. Despite not having a single drug on the market, Moderna is valued at over $7.5 billion –demonstrating the enthusiasm for these strategies.
A lot of this interest in RNA therapy is driven by the possibilities of better vaccines. Researchers are working on using RNA-encoding proteins from pathogens, like influenza, rabies, or Zika to develop vaccines. After receiving the new mRNA, cells translate the strands into protein, stimulating the immune system to produce a response specific to the exact strain of the pathogen.
This would eliminate current vaccines' need for an injection of live, dead, or weakened pathogens. Some researchers are even now applying this concept to cancer. Like viruses, tumors have specific signatures, called neoepitopes, that immune cells can recognize and then fight. Initial cancer treatments using mRNA to target neoepitopes have shown some clinical success, and at least six companies have been founded to pursue this approach.
The short, specific arrangement of nucleic acids make RNAi easier to manufacture than more complicated protein-based therapies, like immunotherapies. Longer strands, like mRNA, are slightly harder, but the investment in their manufacturing is not scarce.
This means RNA therapy will likely be cheaper, more stable, and more accessible than complicated, personalized gene-based treatments.
“If we can stimulate the immune system efficiently in vivo, that would have huge value in lowering cost,” says Hammond. (And Moderna’s mRNA cancer vaccine candidates are aiming to do just that.)
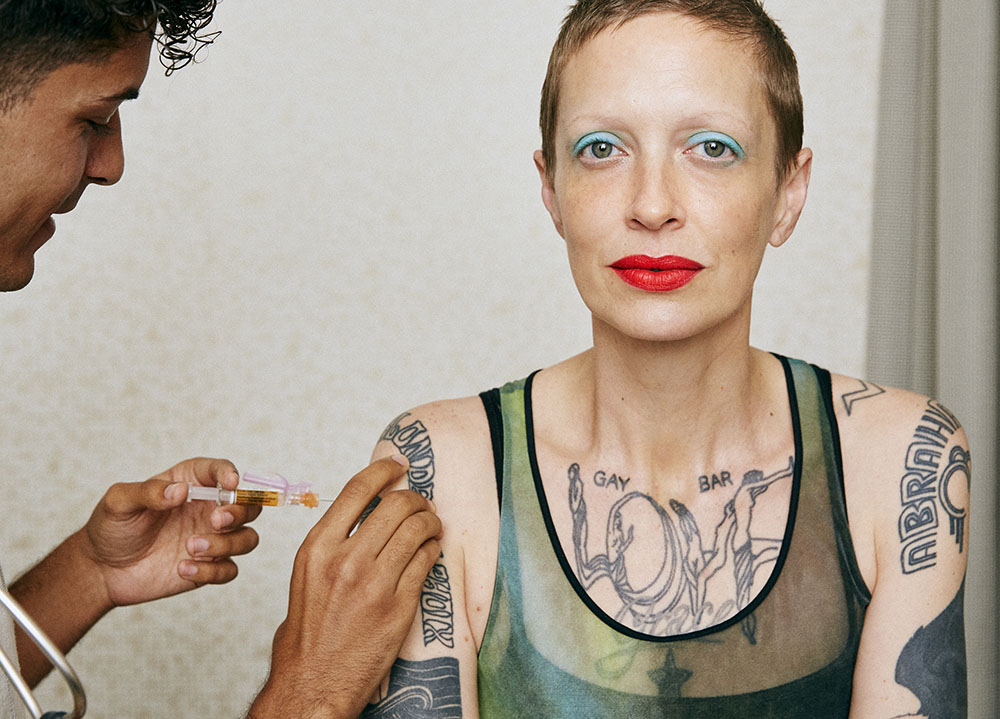
Heather Hazzan, SELF Magazine
Of course, there are still a few challenges to be worked out: as in gene therapy, delivery remains a primary issue. Both RNA and gene therapy need to be delivered into the cell, through one of many potential solutions. (So far, virus vectors and lipid nanoparticles — a ball of fat-like molecules – remain frontrunners.) There's a growing variety of delivery strategies — with patent battles unfolding. For the field, this is a confident sign that profitable, proven methods will be found to deliver RNA to the cell.
“Having all of these [therapies] out in the market at once will accelerate the use of all of them. They are going to synergize each other,” says Hammond.
But after a decade of painstaking progress, RNA therapies are finally poised to become a widely-applicable approach.
“We can now move back and forth along the central dogma to tackle diseases,” says James Kaczmarek, a senior PhD candidate in the Anderson Lab at MIT who has chronicled RNA therapies extensively. “Engineering efforts over the past decade have brought the field to clinical reality."
It's difficult to overstate the potential impacts for medicine.