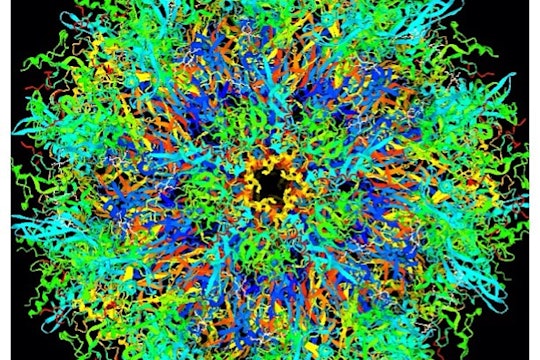
Cryo Mariena / Flickr
Could a virus with a sweet tooth become a weapon against cancer?
Researchers harnessed a virus' natural behavior to attack tumors
Historically, bacteria, parasites, and viruses have been considered dangerous because they spread infectious diseases. But scientists have started to try to harness pathogens for beneficial purposes. Researchers have discovered ways bacteria in our gut help us digest food, and are even trying to develop certain bacteria and parasites as medicines for a range of disorders.
But unlike bacteria and parasites, viruses aren't actually living cells. They must infect and exploit a living cell to stay alive and reproduce, so it's hard to imagine how to turn them from foe to friend. But recently, researchers from Umeå University in Sweden identified a virus that is very choosy about what types of cells it invades. As it turns out, it prefers cancer cells.
It's an adenovirus, which are a common group of viruses composed of a clump of DNA encased in a protein coat. Decorating the coat are fiber proteins that the virus uses to attach to specific molecules on its cellular target. Once attached, the host cell ingests the virus and ruptures its protein coat, expelling the viral DNA inside the cell. The viral DNA then supplants the cell’s previous genetic program, instructing it to manufacture more viruses. Eventually, these cause the bulging infected cell to burst, unleashing freshly-minted adenoviruses that seek out other cells and spread the infection.
Adenoviruses at large
What makes this particular adenovirus, called HAdV-52, special is its two distinct fiber proteins. Most adenoviruses have one type of fiber that recognizes protein receptors found on many cells throughout the body. As a result, they can infect cells relatively indiscriminately. But HAdV-52 also has a second, shorter fiber that doesn’t bind a protein at all. In 2015, the Swedish researchers reported that this second fiber binds to sialic acid, a type of carbohydrate that adorns many cell surface proteins.
Many forms of sialic acid are found throughout our cells, but certain forms are particularly rare. Like other carbohydrates, sialic acid exists in polymer chains of varying length and shape. The shape of these chains is important: Consider starch and cellulose, two common polysaccharides of glucose. Our bodies can easily break down starch into useful glucose units our muscles can use, but cellulose passes through our digestive tracts unaltered. The critical difference between them lies in which linkages are recognized by our proteins.
The researchers wondered if HAdV-52's short fiber could likewise discriminate between different forms of sialic acid on cell membranes – in their 2018 study, they took the short fiber protein and exposed it to a synthetic array of different forms of sialic acid in order to identify which form it prefers. They found the strongest interaction with a single unique linkage of five or more sugar units called polysialic acid (polySia).
During human brain development, polySia is abundant in neural cells, and after maturation, it's only found in regions undergoing the formation of new synapses. But it turns out that some lung and brain tumors also express polySia – and its detection is associated with a poor prognosis. To confirm the finding from the array in a cellular context, the researchers exposed the short fiber protein to two different cell lines from a single cancer patient. Both cell lines had sialic acid on their membranes, but only one had polySia. The cells which had polySia bound to the short fiber more than five times more strongly than the cells lacking polySia.
An electrostatic whip
PolySia is like an electrostatic whip that can disrupt nearby membrane proteins, such as those involved with anchoring the cell in place. Cells throw negatively charged polySia on their membranes for a specific reason: to detach from a rigid matrix of cells and migrate somewhere else. This explains polySia’s function in regions of the brain that are rearranging to form new neural architecture, as well as in metastatic cancer cells that are detaching from their primary tissue. Ironically, HAdV-52’s short fiber forms a tight interaction with polySia, a molecule that repulses pretty much everything else.
When the researchers examined how the virus' short fiber and polySia interacted using a technique called nuclear magnetic resonance, they found the explanation for why HAdV-52 prefers this form of the sugar. Only the last sialic acid unit fits into a binding pocket on the short fiber, while the other units in the chain stick out along a positively-charged surface of the viral protein. Molecular modeling simulations suggest the other sialic acid units form electrostatic interactions with this surface, stabilizing the link.
Virologists appreciate the new atomic-level understanding of HAdV-52’s affinity for polySia, but almost everyone else cares more about its applications: can it be repurposed as a cancer-fighting tool known as an oncolytic virus? A tumor-targeting virus has already been FDA-approved for the treatment of melanoma, but it required genetic manipulation of the virus's fiber protein to direct it at tumor cells. In contrast, HAdV-52 could potentially be used as is, although further research is needed to prove its safety and efficacy in lab animals before being tested as a human cancer treatment.
Other recent advances in cancer treatment have similarly used technologies that exploit particular features of cancer tissue. For instance, the recently-approved immunotherapy CAR-T is proving to be transformative for patients with aggressive blood cancers. But solid tumors pose larger obstacles for CAR-T, in part because they cloak themselves in sugar molecules that shield them from drugs and immune cells. Therapies based on HAdV-52 could turn those sugars against the cancer – a potential game changer for treating the kinds of cancer with the worst outlooks.
As cancer treatment paradigms evolve from small molecule drugs to more exotic processes, keep an eye out for this kind of cancer-fighting virus.
I’m wondering if anything is known about potential off-target effects? You mention that PolySia is naturally found in developing brain regions. Is it thought that the blood-brain barrier will protect the engineered virus from interfering with this development? And is PolySia naturally found in other tissues undergoing reorganization?