Everything grows – that's how it goes – but the 'hows' and 'whys' will fascinate you
Fruit fly eggs are revealing clues about how we grow
It seems intuitive: things are born, and then they grow. It's a marker of passing from infancy to maturity, through all the steps in between. But dig a tad deeper, and it turns out that the way growth works is complex and interesting: there is a multitude of mechanisms that regulate the whens, hows, and to-what-extents of growth.
And while there's still a lot to learn, we are making strides in our understanding by looking in the unlikeliest of places - insects.
Questions about growth regulation come in two flavors: the absolute size or the relative size of a structure. I find myself more interested in how size differences emerge – the relative size question – and I am not the first.
As early as the 17th century, Galileo correctly observed that humans do not grow to their adult size through uniform expansion. Rather, we achieve our final size and form through relative or differential growth: during postnatal development, some parts, like our limbs, grow faster than others, like our heads.
About 200 years later, D’Arcy Thompson and Julian Huxley joined and advanced the discussion. Thompson remarked on the seeming inevitability of relative growth, a phenomenon so complex that uniformity would be an “unlikely and an unusual circumstance.” But, he added, “rates vary, proportions change, and the whole configuration alters accordingly.”
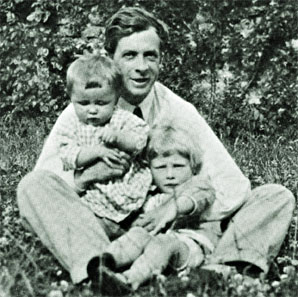
One large and two small Huxleys
In 1936, Huxley coined the term “allometry” in a published study of giant fiddler crab claws, a term which has since been used to describe the mathematical relationship between the sizes of deer antlers and their bodies, and the sizes of our hearts or brains and our own bodies. Researches have since followed up on this work, but the mechanisms driving these differential growth patterns have remained mostly unclear.
What spurs relative growth?
I decided, in grand research tradition, to go back to basics to learn more about how growing works. After all, by studying simple organisms, scientists before me had succeeded in drawing out sophisticated insights into how it all works.
Fruit flies, for example, have two sets of flight appendages: the usual wings, and a smaller pair of club-like appendages, known as halteres, that are used for balance and steering the fly into your salad vinegar. While wings are comprised of about 50,000 cells, halteres only have about 10,000. This discrepancy in cell number – and hence, in size – can be explained by differences in gene expression between the two structures. This example highlights one potential mechanism for size control: pre-patterning – the idea that the roadmap for the structure's development is genetically encoded in the cells themselves.
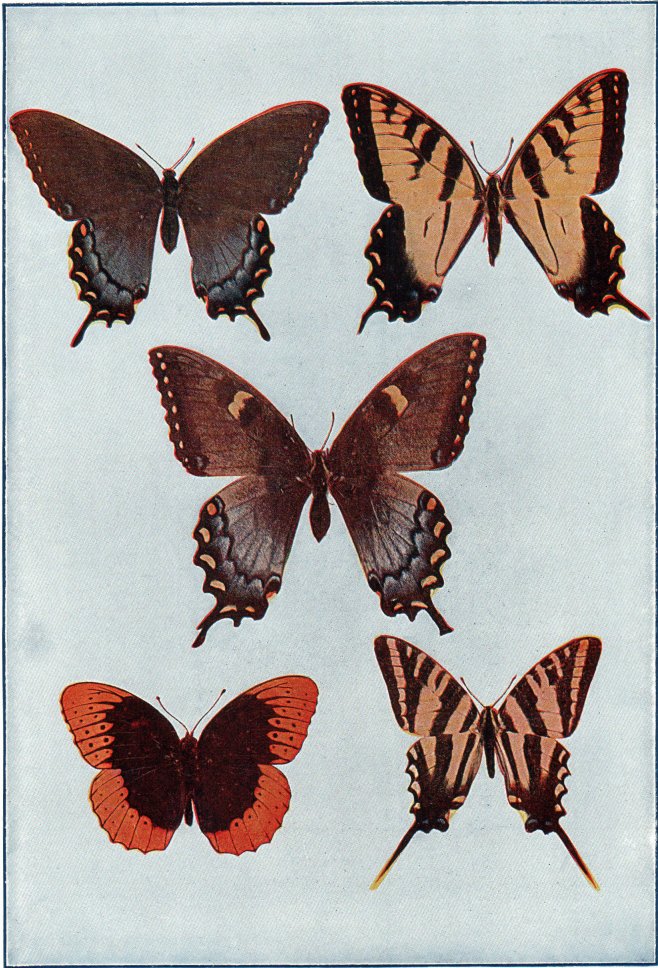
It's not a contest
Butterflies have different wing sizes for seemingly different reasons. These wings also develop from specialized patches of cells in the butterfly larva, and they do so once the larva has stopped feeding. Biologists H. Frederik Niihout of Duke and Douglas Emlen, now at the University of Montana, elegantly showed in a 1998 paper that size differences between the two sets of growing wing appendages can be accounted for by competition for resources that the larva acquired before it had stopped feeding.
This was best exemplified when researchers removed the cells that give rise to the hind wings: in such butterflies, the authors noted a compensatory increase in the size of the forewings, presumably due to greater availability of resources.
Fruit fly wonders
I've been studying fruit fly egg chambers to understand even more about how different parts of a structure grow at different rates and to different sizes. The egg chamber is a small, multicellular structure that holds a single egg in the female fruit fly, and nearly every aspect of this structure is a marvel.
Well-fed and reared female flies are incredibly prolific at egg production, laying anywhere from 50 to 150 egg chambers a day during most of their adult life. A human woman would have to give birth to a full-term child every three hours if she is to reproduce at an equivalent rate by weight. Egg chambers grow in "assembly lines" that order them temporally from youngest to oldest, and each egg chamber can be extracted, fluorescently tagged, and visualized.
Not the wondrous eggs you're looking for
Furthermore, the 16 cells that make up the egg chamber arise from a single founder cell that undergoes four synchronous and incomplete divisions. These divisions leave the cells connected through intercellular bridges, called ring canals, that allow for transport and exchange among cells in the cluster, and allow us to uniquely identify each cell. This is largely because one cell is specified as the egg, while the other 15 are designated nurse cells. True to their name, these nurse the egg by providing it with the RNAs, proteins, and organelles required for its development.
In short, the egg chamber is the stuff of dreams in the world of simple multicellular systems: in addition to its simple and stereotypic structure, we can study most of these processes with single-cell resolution, while witnessing collective behaviors that emerge from the interactions of its multiple cells.
It is also an interesting structure. Although the egg chamber grows by four orders of magnitude in three days, it does so non-uniformly: the 16 cells are not of the same size at any point throughout the egg chamber’s development. But what was the pattern of cell sizes? How did it emerge? My PhD advisor, Princeton chemical and biological engineer Stanislav Shvartsman, was asking these questions when I grew curious, too.
Egg bias
So over a few months in my fifth year as a graduate student, we collected and fluorescently labeled a few dozen egg chambers at various stages of development. Using three-dimensional images, we then identified each cell in each egg chamber and measured its size. This allowed us to quantify the spatial pattern of cell sizes that emerges within the cluster as it grew.
Our data showed that distance to the egg, defined as the number of ring canals separating each cell from it, is the primary factor affecting cell size. Four cells are one edge away, six are two edges away, four are three edges away, and one cell is one edge away. Four groups of nurse cell sizes thus emerge.
We hypothesized that this pattern relied on the unequal portioning of material within the cluster and proposed a mathematical model based on the idea that transport of material is biased toward the egg. This model, despite its simplicity, correctly predicted the experimentally observed pattern of cell sizes and the emergence of four groups of nurse cell sizes.
Perhaps most interestingly, the model revealed the allometric growth of cells, a feature usually associated with much larger systems. That is, we could mathematically relate the sizes of pairs of cells at different distances from the egg to each other through a power law relation - one not unlike that proposed by Huxley to relate the sizes of fiddler crab claws to the sizes of their bodies.
Perhaps the egg chamber can't tell us why our head and limb proportions are what they are, or why we stop growing. But this basic science brings us one step closer to uncovering mechanisms of differential growth that are at play at various times and locations, separately and in coordination, throughout our development.
With a phenomenon so complex, scientists are constantly on the lookout for simple systems that can be visualized, that can be modeled, and while small, can be put to use to answer big questions. The fruit fly is one such animal, and the egg chamber is clearly one such system.